Chapter 8: Vascular plant anatomy: primary growth
As described in Chapter 6 the three organs of vascular plants (roots, stems and leaves) have the same basic structure: a boundary of dermal tissue enclosing ground tissue that has one to many strands of vascular tissue running through it. The three organs differ in the distribution of vascular tissue: in roots it occurs as a single central strand; in stems, the vascular tissue occurs as multiple bundles imbedded in ground tissue; and in leaves the vascular tissue often occurs as a reticulate network of veins or as parallel strands of vascular tissue. In both cases there is ground tissue filling the space between vascular strands and dermal tissue. This basic anatomy is easily seen in asparagus if one trims the base and looks at the cut end. The dermal tissue is the tougher outside, the vascular bundles are seen as small circles scattered in the outer portion of the stem, and ground tissue makes up the rest.
Roots and shoots show two polarities, a radial polarity, meaning that tissues and cells differ as one moves outward from the center (along a radius), and a proximate/distal polarity, meaning that cells at the tips of organs, where they are produced, differ from cells away from the tip, cells which are older. Leaves have a tip to base polarity and often have a top/bottom polarity.
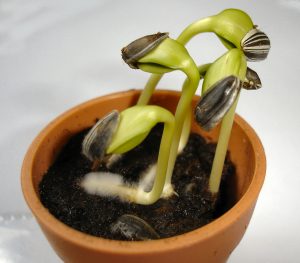
In this chapter, we describe in more detail the plant anatomy of flowering plants resulting from primary growth (growth derived from root or shoot apical meristems), and consider the developmental changes and consequently the patterns shown with age (distance from the apex).
TOPICS
- Root development
- Mature root anatomy
- Shoot development
- Mature shoot anatomy
- Leaf development
- Leaf anatomy
Root Development
If a root is sectioned along the long axis (i.e., a longitudinal section) its developmental pattern is readily apparent. Near the tip is the meristem, recognized by the small size of cells and by mitotic activity, often evidenced by the appearance of chromosomes. Moving back from the tip (proximally, towards the plant body) one encounters older and more mature cells, recognizable because they are larger, no longer dividing, and possess features that distinguish different cell types, e.g., secondary cell walls of tracheids and vessel tube members. Because there is no more cell division or expansion after one moves a short distance from the root apex, the diameter of a root showing only primary growth is generally constant along its length, except for the terminal few millimeters. (Roots exhibiting secondary growth do increase in diameter and are discussed in the next chapter). Cells that are produced by the root apical meristem expand the most in a distal/proximal direction (up/down, assuming the root is vertical) and produce cells that are elongate in this direction. There is much less expansion radially, hence roots primarily grow longer, not wider, and this growth occurs near the root tip. Even more significant than the expansion of individual cells is the fact that most cell divisions in the meristematic zone divide cells, so that most of the additional cells that are produced are in the longitudinal (distal/proximal) plane. This is similar to how a unicellular filament divides to extend itself, with cell divisions that are perpendicular to the long axis of the filament. Cell divisions in the root apical meristem adds cells in the distal direction and only to a limited extent do roots add cells radially. Most roots are roughly 20 to 100 cells wide (assuming only primary growth) but roots are often millions or trillions of cells long.
Assuming a cell division that adds to the number of cells in the distal/proximal plane, a second key consideration following cell division is whether the cell that remains meristematic (and does not grow) is distal (towards the tip) or proximal (towards the rest of the plant). In the vast majority of cell divisions of root meristematic cells, the cell that remains meristematic is distal, and the expansion of the other cell pushes the meristematic region into the soil. However, in a small portion of cells it is the proximal cell that remains meristematic and the distal cell matures and becomes part of the protective root cap, located at the extreme distal end of the root. The cells of this root cap are continually sloughed off as the root extends through the soil, and the root cap ensures that meristematic cells themselves are not sloughed off.
Developmental changes in primary root growth
Proceeding proximally from the root tip one encounters the following regions which transition gradually and overlap:
- zone of cell division, the embryonic region, often less than one millimeter
- zone of cell expansion, generally only a few millimeters in extent, a region where cells are elongating, and to a much lesser extent, getting bigger in diameter; .
- zone of cell maturation, a region where cells develop characteristic features. This zone extends from less than a cm to several cm in length. In the youngest part of this zone root hairs are produced but they soon senesce and are lost from the plant
Significant aspects of cell maturation zone include (in order from the tip as one moves proximally):
- conducting elements of the phloem become functional
- the waterproof compound suberin is deposited as a casparian strip
- conducting elements of the xylem become functional
- root hair appearance and disappearance. Root hairs are extensions off of dermal cells. They are produced after these cells have stopped elongating but are present for only a short time before senescing. Hence, root hairs are only present in a relatively small section of the root.
The significance of the casparian strip
These hydrophobic deposits initially occur as a band that blocks water movement through the wall from the outside to the inside. Eventually the entire endodermal cell wall is coated. The casparian strip forces water and any minerals dissolved in water to enter the cytosol at some point in their journey between the soil and the xylem tissue. Before the casparian strip is deposited, i.e., in the youngest part of the root, water can move from the soil to the center of the root through the ‘apoplast’, a term that describes the collective space of cell walls and any water filled spaces between cells, which typically includes at least 10% of the tissue volume. Because the endodermal cells are tightly bound to each other, once the casparian strip is deposited water is forced to move through the symplast in order to cross the endodermis and get to the interior of the root. The symplast is a term that describes the collective volume of the cytosols of all cells, collective because all cells are interconnected by plasmodesmata, membrane bordered cytoplasmic threads that run between cells.
The casparian strip of the endodermis, once deposited:
- allows the plant to regulate, by virtue of the selective permeability of cell membranes, what minerals do and do not enter the xylem tissue, the conduit to the top of the plant
- allows the plant to, under certain conditions, concentrate solutes in the root xylem because the apoplast solution inside the endodermis (and connected to the xylem tissue) is separated by a two membranes (one providing entry into the symplast, one providing exit from the symplast) from the apoplast solution outside the endodermis. Note that the apoplast outside the endodermis is continuous with the soil.
- decreases the ease with which water can move from the soil to the root xylem.
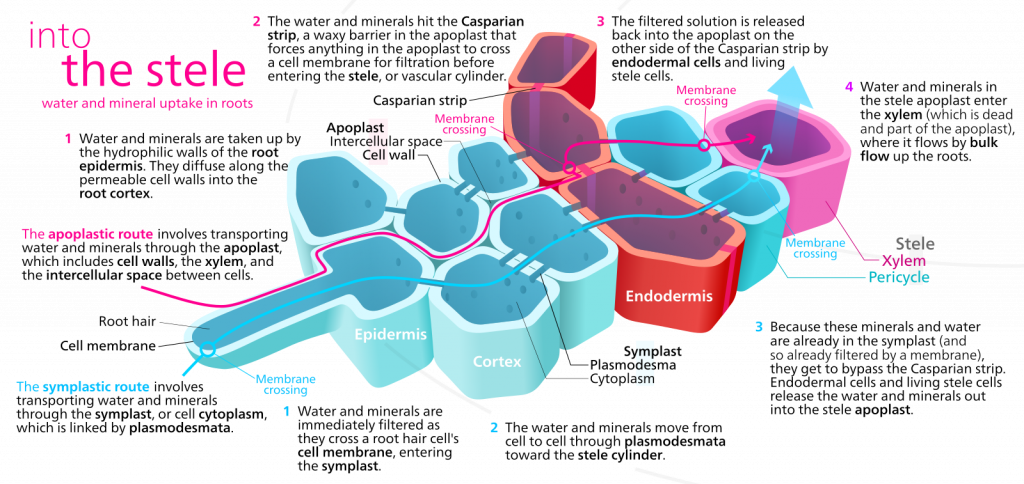
Role of root hairs
Root hairs appear when of the epidermal cells produce a thin outgrowth, called a root hair, that extends perpendicularly from the root into the soil. The root hair is thin (~ 10 um) but may extend several mm into the soil. As discussed earlier, although root hairs greatly increase the area available for water and nutrient absorption, their more significant effect might be in increasing the volume of soil within certain distance to the root. Considering root hairs, water/nutrients may:
- enter the cytosol of the root hair and then proceed inwards through the symplast
- enter the cell wall of the root hair and move through the apoplast to the interior of the root (note that once the casparian strip is deposited in the endodermal cell walls, this root is blocked)
- by-pass the root hair and move through the soil to the root proper
For moist soils water may move most quickly through the soil rather than using the routes through the root hairs and it is possible that root hairs may be most significant in nutrient absorption rather than water absorption
Mature root anatomy
A typical root cross section shows dermal tissue on the outside, surrounding a region of ground tissue (the cortex) which surrounds the endodermis, recognizable because the suberized layer of the cell wall picks up stain. Just inside the endodermis is the pericycle, a ring of parenchyma cells which can be stimulated to form root apical meristems that grow out of the root to form lateral roots. Inside the pericycle is the vascular tissue which is arranged differently in different roots. Some roots have a central pith of parenchyma cells while most roots have a central, solid core of vascular tissue.
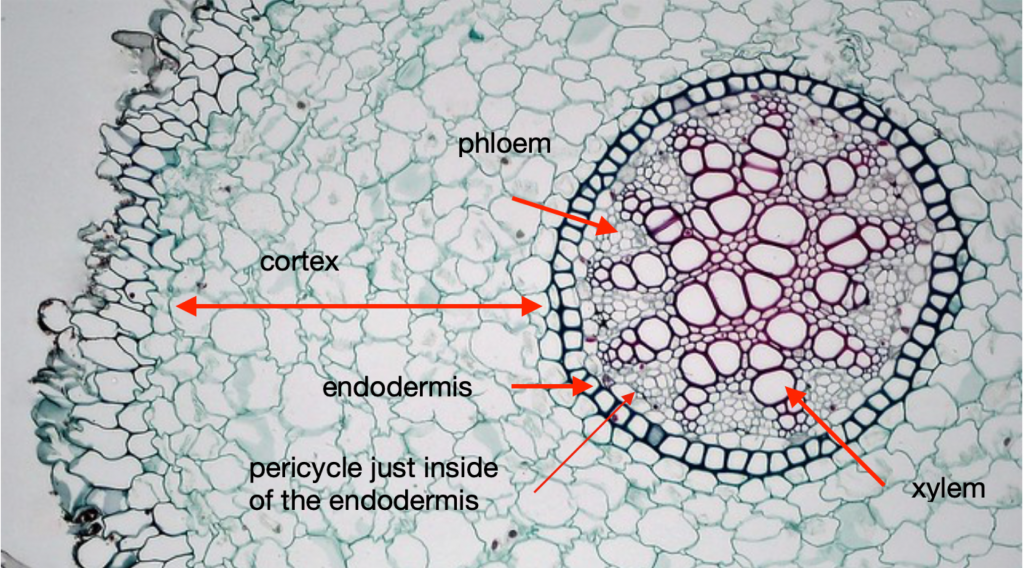
Shoot Development
The basic pattern of development for shoots is the same as that for roots: a terminal region of cell division above a region of cell growth above a region of cell maturation. But shoot growth is more complicated than root growth in several ways, one is the fact that shoot apical meristem not only extends the stem but it also produces embryonic leaves (leaf primordia) and branch shoots (bud primordia) positioned just above the leaves. The presence of these structures divides the stem into nodes, the places where leaves connect with the stem, and internodes, the spaces between nodes. Both of leaf and bud primordia develop vascular tissue that needs to be connected with the vascular tissue of the main stem. If one were to follow the vascular tissue in a leaf or a branch back to the main stem, one would observe one or more bundles of vascular tissue extending from the leaf/branch to the stem. This accounts for the presence of ‘vascular bundles’ in the stems of flowering plants: fundamentally they represent the traces of vascular tissue running to the leaves and branches. At the nodes one can see vascular traces diverging from the stem to enter leaf and branch primordia. Ferns, horsetails, clubmosses and a number of fossil plant groups have different patterns of vascular tissue distribution within the stem (described as ‘stelar structure’) and, because vascular tissue is often represented in fossils, its distribution has been useful in classifying fossilized vascular plants.
Leaf primordia are produced in a characteristic pattern that depends upon the species of plants. The most easily described pattern is one where leaves are produced in pairs on opposite sides of the stem. Sequentially, leaves are produced in pairs occur ring with a 90o rotation from the previous pair of leaves, i.e., if you were viewing a stem from the top and the first pair of leaves were north and south the next set of leaves would be east and west. The next set of leaves produced would be back to the original north-south orientation. Most plants have a more complicated phyllotaxy, i.e., arrangement of leaves, that can be described by counting the number of new leaves and the number of rotations around the stem before you end up with a leaf directly under another leaf.
While the elongation of roots, brought about the expansion of newly produced cells, is generally consistent in time and space, occurring soon after cells are produced and very close to the root apical meristem, elongation of stems is not as consistent. Elongation of shoots results from extension of the internodes. In some plants, internode growth occurs close to the shoot tip, resulting in a stem with leaves that separate from each near the tip of the shoot and at a pace consistent with the production of new leaf primordia. In other plants internodal growth is delayed or absent, producing very short stems with multiple leaves very close together (rosettes). Such a structure may be permanent or may be temporary until a particular cue is received and the stem ‘bolts’, rapidly elongating by increasing the space between leaves, e.g., in lettuce or spinach. Some monocots (see below), in particular grasses, have meristematic zones at the nodes of stems and at the base of the blade of the grass leaf. These meristems are activated if the stem or leaf above them is damaged (usually from grazing).
In contrast to roots, there is no maturation of cells on the distal side of the shoot apical meristem, i.e., there is no ‘shoot cap’ (cf. the root cap) derived from the shoot apical meristem. Generally, the this apical meristem is not being pushed through the soil so the function that the root cap provides is not generally required. However, the shoot apical meristem is covered by young leaves that grow very close to the tip of the shoot and are able to cover it. Some stems, called rhizomes, do grow through the soil and they are protected by modified leaves called cataphylls that protect the shoot apical meristem and form a pointed structure that can more easily be pushed by growth through the soil. Similar modified leaves, called bud scales, cover the shoot apical meristem of woody plants during the extended periods when they are not growing. While most roots often are actively growing most of the year including during the growing season, shoot growth for plants in seasonal habitats is often of much duration. Many plants, especially trees, exhibit growth (extension of stems) for only a short period, often less than 30 days in the spring. However shoot cell division occurs much earlier than cell growth, often as much as eight months earlier, that is some new cells expanding in the spring were produced in late summer of the previous year.
Mature shoot anatomy
Traditionally, flowering plants were separated into two groups, monocots and dicots, based on a number of features, one of which was stem anatomy. While the monocot group is still considered to be a valid phylogenetic entity, most workers consider ‘dicots’ to be an artificial grouping, and have separated dicots into ‘eudicots’ and several other groups. The vast majority of the former dicot group are eudicots and in the discussion below we will use the term dicots.
Dicot stems have vascular bundles arranged in a ring close to the margin of the stem. The tissues running from the outside to the inside are: epidermis, cortex, vascular bundles (in a ring), with variable amounts of ground tissue in between the bundles), pith.
Monocot stems differ from dicot stems in having vascular bundles scattered throughout the stem.
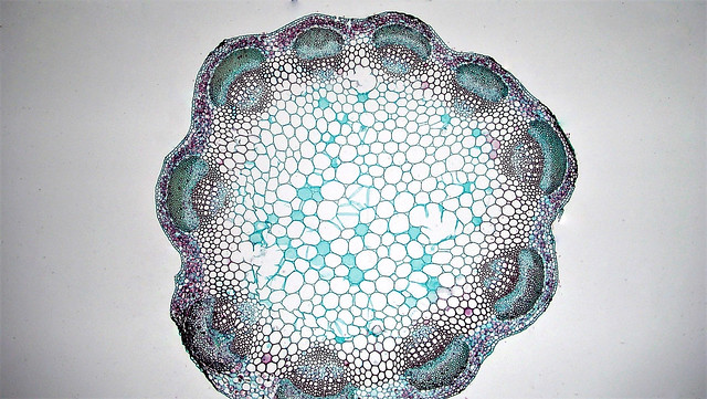
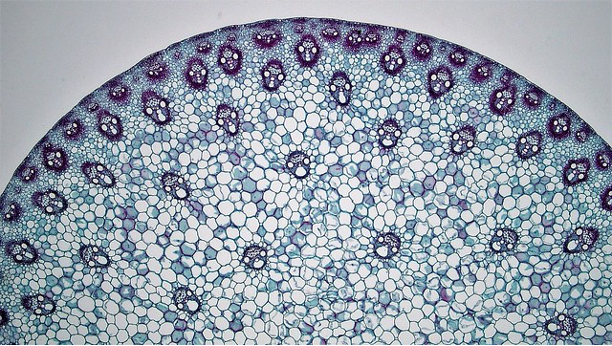
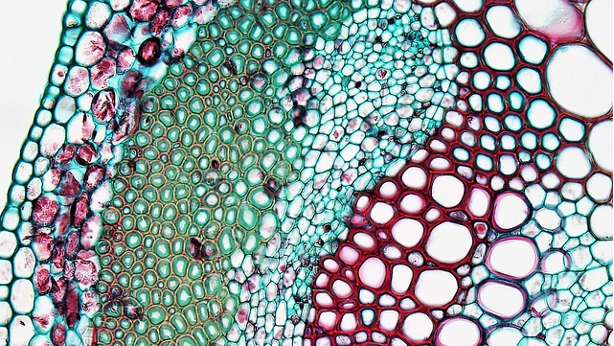
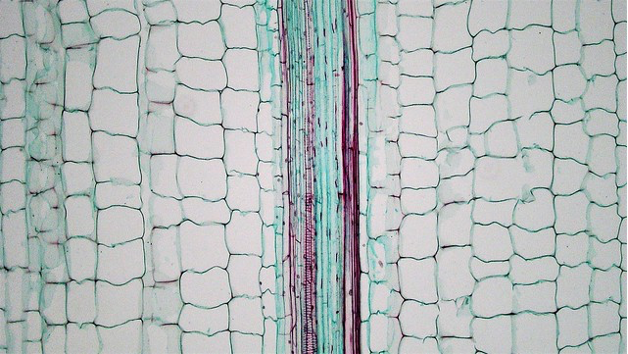
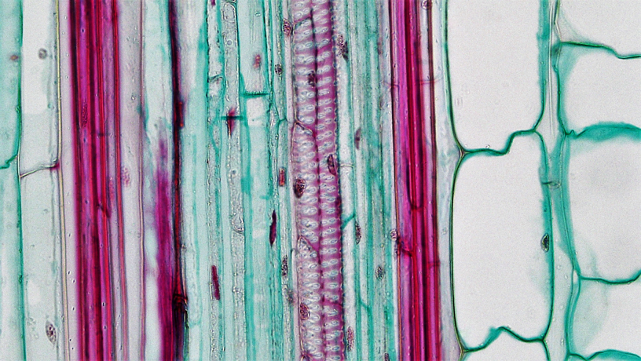
Leaf development
Unlike roots and shoots, leaves are determinate structures whose developmental pattern is comparable to humans and frogs. They start as an embryo called a leaf primordium, whose cells divide and subsequently grow for a defined period of time to produce a three-dimensional form that is often substantially more complex than the cylindrical structure of roots and stems. Moreover, the diversity in leaf form found in vascular plants, especially for flowering plants, far exceeds that found in roots or stems. After cellular division and growth cease, the leaf remains in an ‘adult’ form for a (generally) defined period of time before it undergoes its final developmental process of senescence that ultimately results in the leaf’s separation from the plant (abscission). Leaf senescence and abscission will be discussed later but it is important to emphasize here that these developmental processes are highly significant to the life of the plant.
Intercalary meristems are present in some leaves, in particular grass leaves, and allow a leaf whose tip (distal portion) has been grazed to resume growth and replace lost photosynthetic area. The ability to grow in this manner has been particularly important to the success of grasses.
Leaf structure
Many leaves show a polarity between the upper surface and the lower, with the lower epidermis having regulated pores, stomata, while the upper surface does not. Additionally, the ground tissue of the upper part of the leaf is layered with cells that are oriented parallel to each other (this region is called the palisade mesophyll) while the mesophyll cells of the lower part of the leaf (called the spongy mesophyll) has cells that are not oriented in a consistent way relative to each other and have much larger air spaces between individual cells. Many monocot leaves have veins that run parallel to each other and this means that if you cross section the leaf you are likely to see cross sections of all the vascular bundles. In most dicot leaves the veins run at various angles and a cross section through the leaf is unlikely to show cross sections through any other bundle except the main central vein.
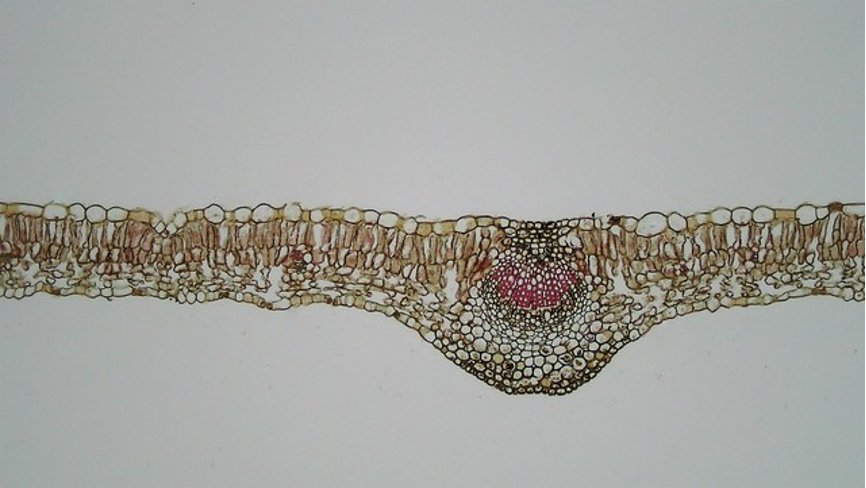
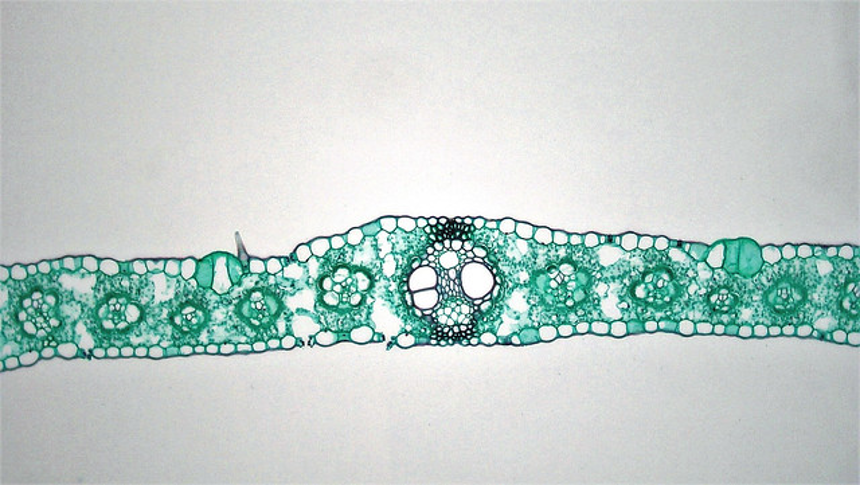
Further Reading and Viewing
See the following sites that were also listed in Chapter 6.
Websites with excellent pictures of seed plant cells and tissues:
- University of Texas Austin Mauseth Lab Table of Contents
- University of Wisconsin Botany Resources
- “See the Plant Kingdom’s Hidden Microscopic Wonders” by Michael Greshko. Nice plant cell (and some algal) pictures.
- Plant Tissues and Organs by the Berkshire Community College Bioscience Image Library. Plant anatomy slides.
- Plant Anatomy Charts by BibliOdyssey. Botanical charts.
Media Attributions
- Sunflower_seedlings © Bluemoose~commonswiki is licensed under a CC BY-NC-SA (Attribution NonCommercial ShareAlike) license
- Into the steele: water and mineral uptake in roots © Kelvinsong is licensed under a CC BY-SA (Attribution ShareAlike) license
- Clover stem © Berkshire Community College Bioscience Image Library is licensed under a CC0 (Creative Commons Zero) license
- Zea © Berkshire Community College Bioscience Image Library is licensed under a CC0 (Creative Commons Zero) license
- Cell close up © Berkshire Community College Bioscience Image Library is licensed under a CC0 (Creative Commons Zero) license
- Longitudinal section of stem © Berkshire Community College Bioscience Image Library is licensed under a CC0 (Creative Commons Zero) license
- longitudinal section © Berkshire Community College Bioscience Image Library is licensed under a CC0 (Creative Commons Zero) license
- Cross section of corn leaf © Berkshire Community College Bioscience Image Library is licensed under a CC0 (Creative Commons Zero) license
- Lilac leaf © Berkshire Community College Bioscience Image Library is licensed under a CC0 (Creative Commons Zero) license