Chapter 18: Matter, Energy and Organisms
One of the activities that define organisms is that at some point in their life, or throughout it, they grow. Growth requires the acquisition of matter and both the acquisition of matter and the incorporation of this material into a living form (i.e., into biomolecules) involves energy. Both matter and energy are needed but it is important to keep in mind that they are two distinct entities that are NOT interconvertible. The energy transformations that organisms carry out involve manipulations of matter but they DO NOT involve converting matter into energy. Energy is obtained by rearranging matter, mostly by converting carbohydrates and oxygen into carbon dioxide and water. What complicates understanding is that matter is needed in two ways (Fig. 1): (1) materially, providing the materials that become part of the organism, mostly carbohydrates or carbohydrate derivatives, (2) energetically, because energy can be made available as matter is rearranged, e.g., converting carbohydrates and oxygen into carbon dioxide and water. Although matter is being rearranged, it is not being transformed into energy.
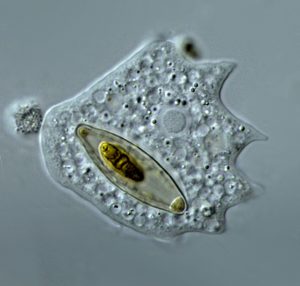
Matter, Energy and the Laws of Thermodynamics
Matter and energy are key players in the process of life at all levels: cell, organism, and ecosystem. Both matter and energy are familiar ideas, yet misconceptions are common, especially about energy and the interplay between matter and energy in biological systems. Matter is straightforward: it has mass, occupies space and can be categorized into elements (e.g., carbon, hydrogen, oxygen) that often are usually present in specific mixtures termed molecules (e.g., carbon dioxide, glucose) that have a characteristic composition of elements and are arranged in specific ways. Living things are made of matter and have a characteristic material composition, being made of biomolecules such as proteins, carbohydrates, and nucleic acids. Life may be partly defined by the ability of living things (organisms) to acquire matter and incorporate it into themselves, i.e., to grow. Life can also be defined on the basis of its ability to manipulate matter in characteristic ways that involve energy transfers.
While matter is an easy concept, energy is much more elusive; consider the following:
- Energy has the ability to affect matter by rearranging it or moving it from one place to another.
- Energy is dynamic and the energy associated with a given bit of matter, e.g., a molecule, depends on circumstances; it is a function of the situation matter finds itself in, the speed it is moving, the location that it is in, in particular its position relative to other matter or relative to electric, magnetic and gravitational fields (which are controlled by matter).
- Energy is a property of systems, i.e., an assemblage of matter in a particular place and with specific relationships with each other.
- Energy describes the ability of a given assemblage of matter (a ‘system’) to change the organization of another bit of matter (another ‘system’ or perhaps ‘the surroundings’).
- Energy could cause atoms or molecules to move in relationship to each other, e.g. a chemical reaction, or cause an object to change position in a gravitational field (rise or fall), or cause a charged molecule or object to change position in an electrical field.
- Just as energy can cause movement of matter, the movement of matter (i.e., matter changing position) changes the energy content and allows energy to be ‘transferred’ from one system to another or one molecule to another.
- Energy can also be transferred to material via electromagnetic radiation, waves of electricity and magnetism that are given off by any bit of matter with a temperature above absolute zero (i.e., every bit of matter!!!).
- Electromagnetic radiation is a ‘form’ of energy that is important to all forms of life but especially so for photosynthetic organisms. Electromagnetic radiation has a dual nature and can be described as (1) a rhythm of electric and magnetic fields, a series of waves with a certain frequency and wavelength, moving at a constant speed, the speed of light, or (2) packets of energy called photons. The energy in a packet (a photon) is related to the wavelength of the waves of electricity and magnetism. Note that these photons/waves of electricity and magnetism are able to interact with matter and transform it, thereby transferring energy to the matter.
- Two other concepts related to energy are heat, which can affect matter by changing its kinetic energy, changing the average speed that molecules are moving, and work, which can change the position of objects in a gravitational field, or perhaps concentrate chemicals in a particular spot (chemical work). While both heat and work are connected to energy and are sometimes considered ‘forms’ of energy, they might better be described as interactions between systems or between a system and its surroundings.
A common feature of both matter and energy is that both are conserved, something described in what is known as the first law of thermodynamics. Although modern physics has demonstrated that matter can be converted into energy and it is their collective entity (matter + energy) that is conserved, in biological systems matter and energy are NEVER converted from one to the other and consequently we can consider each to be conserved — there is always the same amount of matter and the same amount of energy. Neither one is created, destroyed or ‘used up.’ The conservation of matter is easily understood, matter can be moved from one place to another, e.g. accumulated in an organism, lost (or gained) by diffusion from (or to) an organism. Elements can be rearranged, e.g. carbon transformed from carbohydrate to carbon dioxide in the process of cellular respiration, but the amount of matter is constant —the same number of carbons, hydrogens and oxygens. Similarly (and much less appreciated) , energy is conserved. It can be ‘moved’ from place to place, or transformed from one form to another (as molecules are rearranged or moved relative to each other and relative to gravitation al, electrical and magnetic fields), but the amount of energy is constant, unchanging. Living systems, non-living systems and combinations of living and non-living systems rearrange matter, and by rearranging matter they redistribute energy. But the first law of thermodynamics states that in all these rearrangements there is a constraint: after any rearrangement, the amount of matter and the amount of energy must be the same as it was in the beginning.
Living things constantly reorganize matter: molecules combine, molecules separate into pieces, molecules move from one place to another. In all of these transformations, matter must be conserved. In addition, the energy must be conserved; consequently, organisms may release energy during some transformations (because the final arrangement of material in the organism has less energy than the initial arrangement); or, if the final arrangement has more energy than the initial one, the organisms must somehow have acquired energy to bring about the transformation.
Since matter and energy play in zero-sum games then one might think that their transformations are rather tedious and potentially circular, with losses in one spot being exactly matched by gains somewhere else, and the potential of ending up exactly where you started. This is not the case, there is a direction to the transformations and it is strictly a one-way flow: you can never return to the starting point. This constraint is dictated by the second law of thermodynamics which states that in spite of the fact that energy is conserved, the amount of energy that can be used to do work is always decreasing. To most, this statement is startling because they assume that all energy can be used to do work; but some energy is not ‘useful’ and the second law states that the amount of ‘useless’ energy is always increasing. The second law of thermodynamics is extremely powerful and this is reflected in the fact that it can be defined in a variety of ways. Fundamentally, its utility rests in the fact that it puts an arrow on rearrangements of matter. Given two possible arrangements, A to B, each with the same amount of matter and energy, the second law dictates that the direction of the rearrangement will always be to a situation that has less useful energy. The second law points out what rearrangements of matter will be ‘spontaneous’, i.e., occur ‘on their own.’ Rearrangements in the opposite direction (the non-spontaneous direction) will only occur if energy, useful energy, is supplied.
The second law adds a second constraint on transformations; not only must matter and energy be conserved but the amount of useful energy must decrease. Consider a system A with a certain amount of matter and energy at a particular time, and the same system, now called A’, a time later; the second law dictates that, barring interaction with the surroundings, the only change in A that is possible as it transitions to A’ is one where there is a decrease in energy available to do work; thus once you leave situation A, you can’t return to it (i.e., get from A’ back to A). A lthough the energy in both is the same, the amount of energy available to do work is diminished as it transitions from A to A.’ This reflects one of the common ways that the second law can be stated: there are no perpetual motion machines. A device can’t get back to where it started without energy from the ‘outside’.
Organisms, matter and energy
How is all of this significant to organisms?? Organisms are defined in part by their ability to grow and since growth requires the acquisition of matter, all organisms need to be able to acquire the specific materials that they construct themselves with. Moreover, growth requires useful energy because work is done in the construction of most new molecules for growth. What complicates understanding is that matter (‘food’) plays a dual role: (1) materially, providing the materials that become part of the larger organism, (2) energetically, providing energy that is made available as matter is rearranged. The transformations of matter and the transfers of energy performed by organisms are intertwined in ways that allow misconceptions to easily be acquired but it is important to remember that matter and energy are two different entities.
But growth isn’t the only reason that organisms need matter and it isn’t the only reason why organisms need energy.
Why organisms need energy
- In addition to needing energy for growth, organisms need energy because they ‘do work’ in a physical/chemical sense. They create electrochemical potentials, they develop pressure, they generate forces that result in movement. Particularly significant is that they perform chemical work as they grow: many biomolecules consist of arrangements of matter that contain more useful energy than the materials these molecules are constructed from, and therefore energy is needed to synthesize them. The process of growth requires organisms to rearrange material, reposition it, in ways that cause the new material to possess more useful energy than what it was made from. This is only possible if organisms have a ‘supply of energy’ and the work that they do is possible because part of the energy in this supply is ‘used’ to allow for the rearrangements of materials. Note that energy is conserved, but the amount of useful energy, the amount that can be used to do work, is diminished.
- But even in the hypothetical situation where an organism is not growing (making more biomolecules) and not doing work (e.g., moving itself or materials within it self), it would still need energy simply to maintain itself. Organisms exist in an organized state that spontaneously degrades to a less organized state. The maintenance of the organized state requires energy. An easily understood example of this involves the charge difference found across the cell membrane, with the inside being negative relative to the outside. This organized situation spontaneously ‘breaks down’ to a less organized one because electrical forces push negative ions out across the membrane and positive ions in. Maintenance of the organized state requires energy because the process of organizing (in this case moving ions across a membrane so they are more concentrated in one place than another) requires energy.
How organisms obtain energy
Organisms’ energetic needs are largely satisfied by acquiring biomolecules (food), generally carbohydrates, and processing them in a group of reactions called cellular respiration. Cellular respiration (Chapter 19) is a controlled ‘burning’ process whereby carbohydrates react with oxygen (the carbohydrates are oxidized), producing carbon dioxide and water. If one compares the energy content of equivalent amounts of carbohydrate plus oxygen to that in carbon dioxide plus water, there is substantially less energy in carbon dioxide plus water. If you burn carbohydrates in a fire the difference in energy is released as heat and light, but in cellular respiration, less energy is released as heat, and none as light, because some energy is ‘captured’ in chemicals, in particular one called ATP. Because the products of the reaction (carbon dioxide and water) are invisible gases many believe that cellular respiration converts matter to energy. But this is impossible, the first law forbids it! The original carbon, oxygen and hydrogen are still present, just now in different places relative to each other. Similarly, the original energy remains but is now present in the ATP that is formed and the heat energy that is released.
Why organisms lose material because of their energetic needs
As an organism carries out cellular respiration it produces two materials (water and carbon dioxide) that are easily lost and sometimes ‘purposely’ eliminated (e.g., in humans, where breathing, i.e., ventilation, facilitates the loss of water and carbon dioxide). As a consequence of cellular respiration, organisms are continually los ing matter as carbon dioxide and water and consequently are also los ing weight. Thus, in order to maintain its weight, a respiring organism must acquire more ‘food’.
Obtaining matter and energy
In order to satisfy their energetic needs, an organism requires a supply of carbohydrates (or other biomolecules) to utilize in cellular respiration. These carbohydrates may be obtained in two basic ways: (1) by consuming biomolecules that have been produced by other living things—carbohydrates or molecules like proteins that can be metabolized to produce carbohydrates or (2) by consuming ‘self-constructed’ carbohydrates that are produced in reactions (usually photosynthetic reactions) that synthesize carbohydrates from carbon dioxide and water. Such reactions utilize ‘sources’ of energy (e.g., sunlight) that allow a chemical reaction to occur where the products have more energy than the reactants. The synthesized carbohydrates are then used to power cellular respiration, i.e., they are converted back to carbon dioxide and water. The group of organisms that consumes carbohydrates that other organisms have produced are termed heterotrophs (hetero-other, troph-eat; literally ‘eat others’), and the group of organisms that make their own carbohydrates to ‘eat’ are termed autotrophs (auto-self, troph-eat; literally ‘self-eaters’). It is important to realize cellular respiration occurs in both groups, they differ only in how they acquire carbohydrates to be oxidized in cellular respiration.
It is critical to keep in mind that matter and energy are two different things but they are intertwined. Energy that is present in carbohydrates and oxygen can be ‘released’ when the material is rearranged in to carbon dioxide and water. The ‘released energy’ might end up as heat, or as work, or in a new arrangement of molecules (e.g. ATP is a rearranged version of ADP plus inorganic phosphate). However, the second law requires that the total amount of energy in the new arrangement (e.g., carbon dioxide, water and ATP) must possess less ability to do work than the earlier arrangement (in this example, carbohydrate plus oxygen plus ADP plus inorganic phosphate).
Appreciate that the ‘food’ that organisms obtain, either by finding it (heterotrophs) or making it (autotrophs) serves a dual function, providing (1) energy (through cellular respiration) and (2) material (through a variety of metabolic pathways where carbohydrates are reconfigured to produce other biomolecules (proteins, fats, nucleic acids). If food provides energy through the process of cellular respiration it is transformed into carbon dioxide and water and these cannot be used materially to make biomolecules. Alternatively, food can provide ‘building materials’ that are used to make more cell membranes, cell walls, cellular enzymes, but this food will NOT be ‘providing energy’. Food cannot provide both energy and building materials at the same time! You cannot ‘have’ your cake (build with it) and ‘eat’ (use it for cellular respiration) it too.
The major topics to be covered in the next six chapters on the growth of organisms are outlined below. As described above, almost all energetic needs of almost all organisms are a consequence of cellular respiration (Chapter 19)—the oxidation of carbohydrates by oxygen, producing carbon dioxide and water. Satisfying the material needs of heterotrophic organisms is a relatively simple story; however it is more complicated for autotrophs, where it involves both photosynthesis (Chapter 20) and mineral nutrition (Chapter 22), the acquisition of mineral elements like nitrogen and phosphorus. Although most prokaryotes satisfy their material and energetic needs in typical heterotroph or autotroph fashion, we will also consider some of the metabolic diversity (Chapter 21) found in some prokaryotes that reveal very different patterns of satisfying energy and material requirements. This diversity is an interesting contrast to the familiar, normal ways of life and also plays a significant role in the nutrition of plants by influencing the availability of certain plant nutrients. We will briefly consider how organisms move materials throughout their bodies (Chapter 24), a process that usually (but not always!) ‘requires energy’. We will also consider the nature of soils (Chapter 23), which serve as reservoirs for the nutrients and water that plants require. A final aspect of growth that we will consider is the rhythms of growth (Chapter 25) that organisms, especially plants, exhibit and how this growth might be modeled.
Media Attributions
- Amoeba © djpmapfer is licensed under a CC BY-NC (Attribution NonCommercial) license