Chapter 30: Threats to agriculture: insects and pathogens
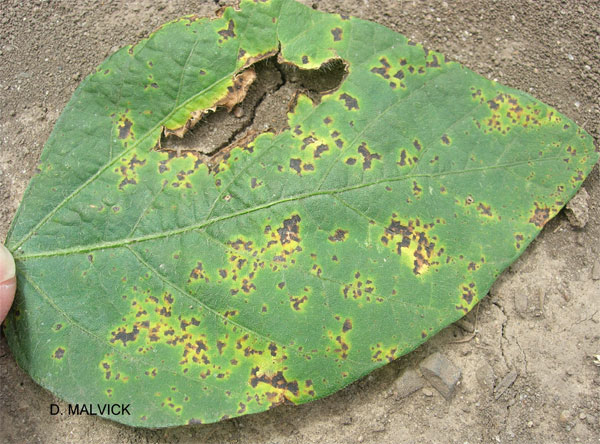
As any farmer will tell you, there are many threats to growing plants. Some of these have been covered in earlier chapters: extremes in temperature and moisture (Chapter 26); and interactions with other plants, i.e. weeds (Chapter 29). A large number of additional threats come from trophic interactions with a vast diversity of grazers, parasites and and predators. Certainly the most familiar of these are insects but other invertebrates (mites, nematodes, mollusks) and vertebrates can be significant obstacles to growing plants. Moreover, a large number of fungi, bacteria (Fig. 1) , protists and viruses are also be important threats.
TOPICS
- Grazing herbivores
- Absorbing herbivores
- Plant defenses
- Boundary
- Chemicals
- Phenology
- A sampling of plant disease
- Phytoplasmas and defining disease
- Late blight of potato—the disease triangle
- Wheat rust disease—complex pathogen life cycles
- Corn smut disease—Mexican truffles
- Dutch elm disease —overreaction can kill you
- Rice blast disease—changing strategies
- Fire blight of apple—bacterial phytopathogens
- Crown gall disease—making a disease a tool
- Viral diseases—tobacco mosiac virus, cauliflower mosaic virus, ring spot disease of papaya
Grazing herbivores
Most animal herbivores, e.g. woodchucks and adult japanese beetles, are considered grazers, eating parts of the plant and using their mobility to get to other plants. However, a substantial number of insect herbivores have feeding stages (generally larvae, e.g. leaf miners, emerald ash borers, larval potato beetles) that are immobile to the extent of getting to new host plants. These might better be considered parasites because they feed solely on one host plant. Most non-animal herbivores (fungi, bacteria) are basically immobile and are considered parasites although some are capable of grow ing from one host to another and many are transported from one plant to another by wind, rain and insects.
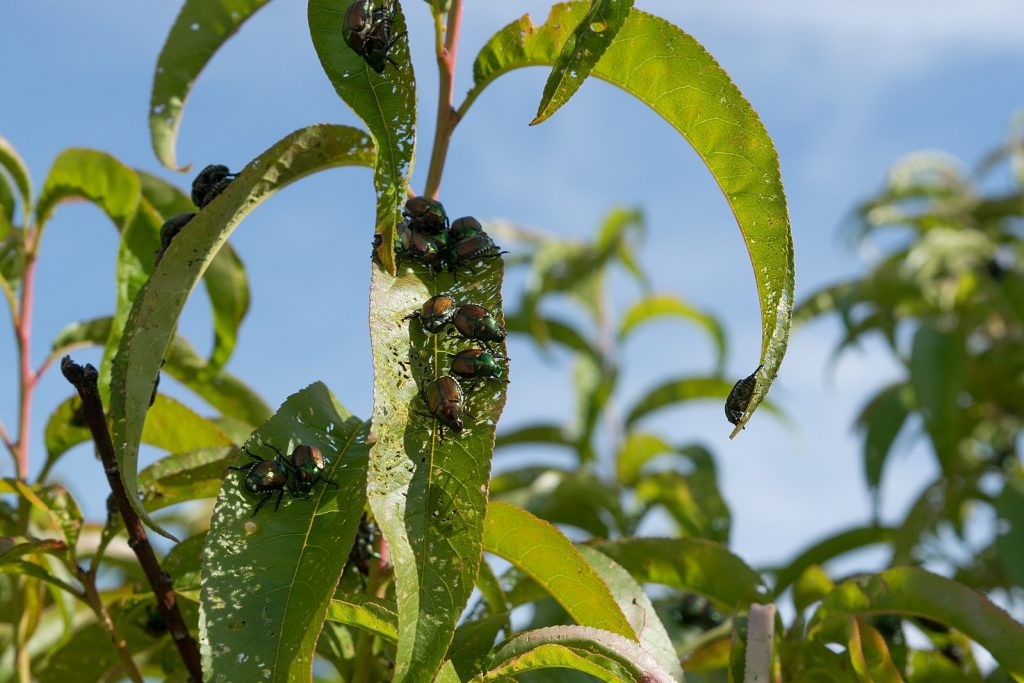
Most grazers on plants are ingesters (see Chapter 27), they remove and consume part of the plant, shred it to some extent and then digest the pieces in an internal tube, and ultimately excrete undigestible to their environment. Grazers, although they can severely reduce crop yields, rarely completely kill the plant it is eating both because they tend to specialize on only part of the plant (most commonly leaves) and because the plant is capable of replacing the organs/parts of organs that have been lost.
The plant material acquired by grazers is generally not very nutrient dense but varies substantially depending upon the part of the plant eaten. Nutritional quality is largely influenced by how many living cells are present (dead cells are basically all cell walls and are indigestible for most herbivores). Most herbivores specialize on one of the tissues listed below, but there are some generalists that feed on multiple tissues, e.g. browsers like moose and deer often eat stems, leaves and meristems.
- primary growth of stems and roots — although stems and roots in primary growth have more living cells than those with secondary growth, they still are nutrient poor. Most of those cells that are living are not very metabolically active and consequently they are poorer in nutrients when compared to cells that are more metabolically active (e.g. leaf mesophyll cells).
- woody stems and roots, heartwood: Heartwood has no living cells and has increased levels of secondary chemicals. It generally has the lowest nutritional quality of any plant tissue. Nonetheless, there are heartwood specialists.
- woody stems and roots, sapwood: Sapwood has some living cells (the rays) and these may be ‘loaded’ with starch and other nutrients, depending on the season. Hence sapwood is considerable more nutritious than heartwood but still is largely composed of cell wall material.
- woody stems and roots, vascular cambium — this meristematic tissue is nutrient rich and has relatively less cell wall material compared to mature cells. Moreover, it is more extensive than apical meristems, i.e. there is more material available to eat.
- the bark tissue of woody stems is nutrient poor with few living cells and an abundance of secondary chemicals. The portion of cells that are living decreases as the stem/root ages and hence bark of younger stems is more nutritious than the bark of older stems.
- stems and roots that have been modified as storage structures — rhizomes, corms, bulbs, stolons. These are composed of living cells that are storing carbohydrates, usually in the form of starch. They are rich in digestible carbohydrates but may be deficient in amino acids (proteins) and other nutrients.
- phloem feeders: These organisms (including aphids, leaf hoppers, mealy bugs and white flies, all significant agriculture pests) are not consuming any cells, but instead tap into the phloem transport system and acquire the materials that are being transported through it. Phloem sap has very high concentrations of sucrose along with much smaller concentrations of amino acids and some minerals. Because the ratio of carbohydrates to amino acids in phloem sap is so high most phloem feeders excrete ‘honeydew’, essentially phloem sap — still rich in carbohydrates but with most of the amino acids removed. Honeydew is utilized by several other organisms, in particular some ant species and the sooty mold fungus.
- xylem feeders: Xylem sap has very few nutrients, almost no carbohydrates but with some of mineral ions. Also, while phloem feeders acquire sap passively because it is under pressure inside the phloem cells, xylem sap is under tension and requires ‘sucking’ (developing greater tensions than already present) in order for it to flow into xylem feeders. Nonetheless, there are some insects (larval cicadas, larval spittlebugs) that feed on xylem.
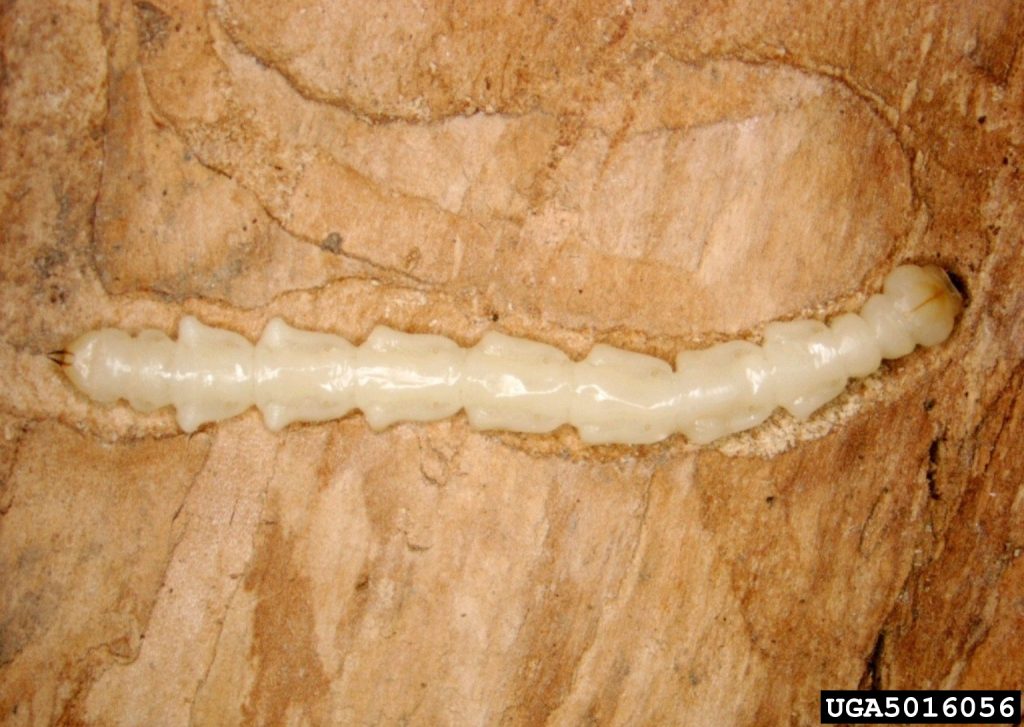
Absorbing herbivores
In contrast to grazers, most non-animal plant herbivores are absorbers (see Chapter 27), absorbing nutrients from ‘their environment’, with ‘their environment’ being the interior of a plant. Most of these organisms are bacteria and fungi, but some, like water molds, are protists and a few are animals (e.g. nematodes, the larval stages of some insects). Most of these organisms are considered parasites and are generally considered to be plant pathogens. They invade plant tissues and then acquire materials in one of three ways: (1) the parasite feeds on materials that ‘leak’ from host cells. (2) the parasite kills host cells, causing cellular materials to become available. Organisms that feed this way are described as being necrotrophic (feeding off dead material). (3) the parasite and host form a structure called an haustorium, a fusion of both the host cell membrane and the parasite cell membrane. (Note that the development of the haustorium generally requires breakdown of the cell walls of both the host and parasite). Materials are transferred from host to parasite through the haustorium and both host and parasite stay alive. Organisms that feed this way are described as being biotrophic (i.e. feeding off living material). Some biotrophs are relatively benign herbivores (they benefit from keeping their host alive), and consequently many acquire relatively small amounts of resources from their hosts. But many biotrophs (e.g. rusts, smuts, downy mildew, powdery mildew) can be devastating to farmers as infestations often can reduce plant yields to next to nothing.
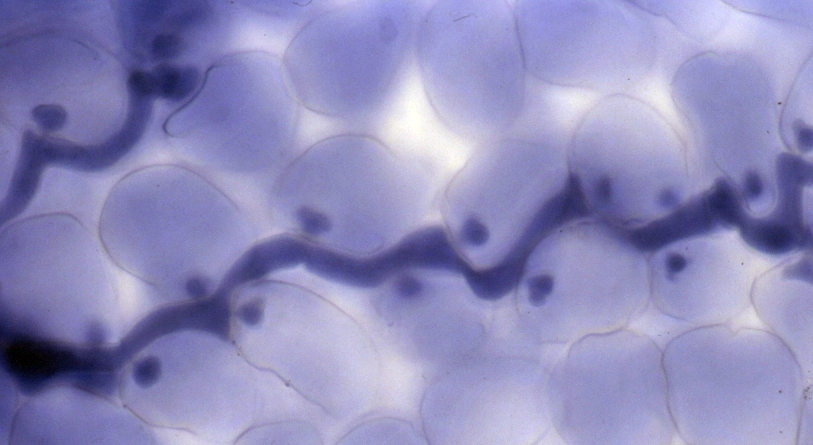
Plant defenses
An effective boundary: Except for large grazing animals, organisms need to get inside plant tissues in order to feed on the plant. The boundary makes this difficult by producing a surface that is hard to penetrate and inhospitable for life (see Chapter 3). For above ground primary growth, the boundary consists of epidermal cells that are tightly bound to each other, making it difficult to penetrate between cells. Additionally, above ground primary growth is coated with a cuticle that is difficult to pierce and hard to live on because it is hydrophobic. Below ground, mature primary roots have an endodermis that serves many of the same functions as the epidermis+cuticle does above ground. However, the youngest parts of roots have not developed an endodermis. This makes water acquisition easier but it also makes it easier for pathogens to enter.
Pathogens/herbivores gain entry both by utilizing stomatal openings and also by having mouthparts that can penetrate the cuticle/epidermis; or having a needle-like or saw – like ovipositer (egg-laying organ); or, in the case of some fungi, producing an appressorium, a specialized cell type that is able to fuse with the epidermis and produce a structure that can penetrate it. Pathogens and herbivores also gain entry through wounds and openings in the epidermis as the result of growth processes, e.g. branch roots and the shedding of leaves and branches.
Chemistry: All plants produce diverse chemicals that influence herbivory and pathogens. Some are feeding deterrent chemicals that are poisonous or may advertise that poisons are present within the plant. At the same time, some chemicals produced by plants clearly attract certain herbivores. The assumption is that these chemicals had once served as feeding deterrents but that the herbivore has developed means to detoxify the poison and is now using the chemical to identify a host that relatively few competitors will be able to utilize. For example most insect herbivores will not eat milkweed but several insects (monarch butterfly larvae, the milkweed beetle and milkweed bugs) choose to feed on milkweed. Some even utilize the plant’s poisons as their own, making them less likely to be consumed.
Plants also produce chemicals because they recognize that they are under attack. The production of these ‘induced’ chemicals imply that the plant has an ability to sense the presence of the pathogen and the chemicals produced may: (1) kill the invader (phytoalexins), (2) trigger defensive responses in neighboring plants and/or attract predators that may control the herbivore, or (3) elicit an ‘hypersensitive response’, causing the invaded tissue to rapidly die. Note that the hypersensitive response may be effective in deterring biotrophic pathogens but actually benefits necrotrophic pathogens.
Phenology: Plants (and farmers) may be able to avoid herbivores/predators by adjusting the timing of seed germination, growth and flower / fruit production.
A Sampling of plant diseases:
Poinsettias, phytoplamas and the nature of plant disease
Poinsettia’s did not always look as most of them do now. The plant in the wild and the plant that was originally propagated as an ornamental plant was much taller plant. It also produced relatively few ‘flowers’ (the structures that look like a flower are actually a cluster of flowers surrounded by colorful, usually red, bracts). Early in the 20th century a plant with a novel form appeared, one that was much shorter, branched much more frequently, and produced more flowers. This form could be propagated by cuttings and the assumption was that the original smaller plant was a mutant, a ‘sport’, like the original naval orange (see Chapter 28). Sports generally cannot be perpetuated by seed because the mutations making them distinctive are often recessive. But they often can be perpetuated by cuttings because cuttings are essentially continued growth of the original plant. There are other phenomena besides mutations that might account for an altered growth pattern that can be perpetuated by cuttings. Two possibilities are viruses and phytoplasmas (small bacteria lacking cell walls that live only in plant cells). Note that all three possibilities for the abnormal plant (mutation, viral infection phytoplasma infection) could be described as ‘plant disease’ but that what is unusual is that in this case the diseased plant is desirable not undesirable. It turns out that almost all the poinsettia produced today are diseased! And the culprit turns out to be a phytoplasma.
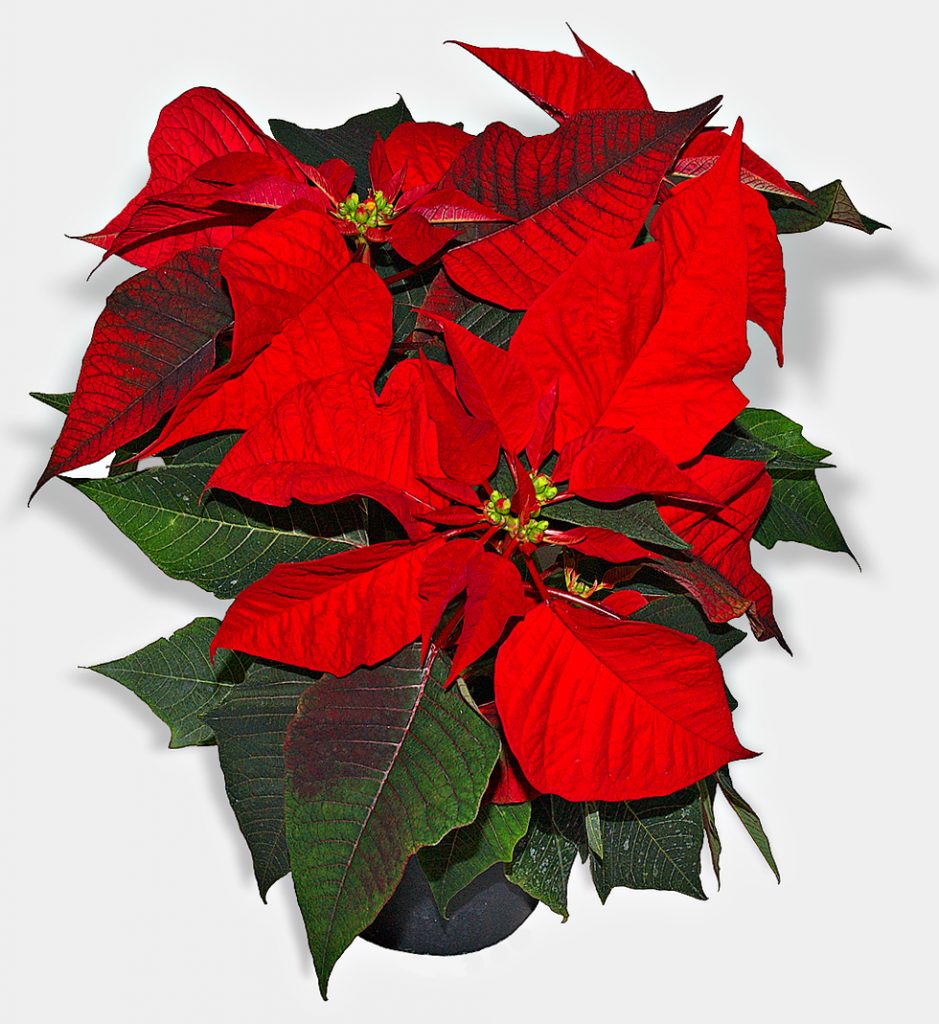
So what is plant disease? In the poinsettia discussion it was associated with ‘abnormal plants’ A difficulty with this definition is that it is ‘normal’ for biological entities to be ‘abnormal’. That is, if you look at a population (group of organisms) they aren’t all alike, they vary, some are ‘outside the norm’. Additionally, ‘normal’ is not readily defined. It can be defined statistically as a central tendency, e.g. abnormal is more than one standard deviation from the mean. Although not perfect, the ‘abnormal’ definition works with plants partly because the most plants under consideration are typically those of economic importance and ‘normal’ is a type of plant that provides the most economic return. Additionally, most crops have been bred to be uniform.
Thus a diseased plant is recognized by abnormal structure or functioning. Common symptoms of diseased plants include stunted or deformed growth and yellowed (chlorotic) or dead (necrotic) leaves. But occasionally one might find a diseased plant that is ‘bigger than normal’, that branches more than normal, or that has leaves that persist on the plant longer than normal. In an arbitrary manner, plant disease generally excludes conditions due to insect herbivores even though they may result in similar effects as ‘true’ plant pathogens. Since this book is dealing with inanimate life we will not consider insect herbivores except as introduced in the chapter on biotic interactions and as covered in the next chapter dealing developing new plants.
Late Blight of Potato and the ‘disease triangle’
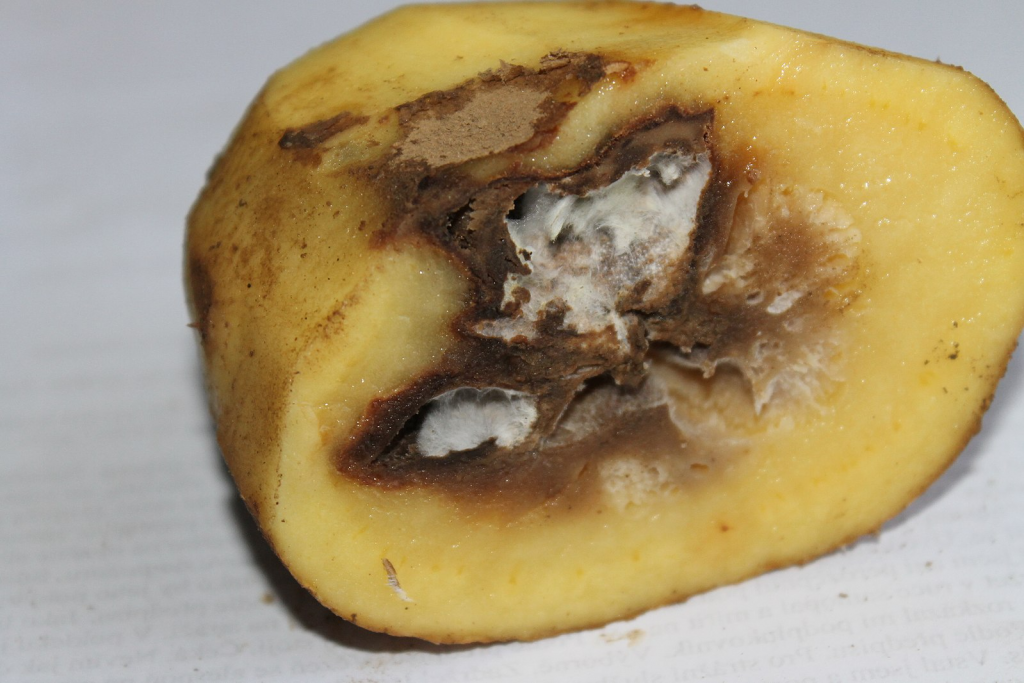
The causes of abnormal functioning, i.e. diseased plants, are legion, including: weather conditions, nutritional (soil) factors, genetic changes and a wide spectrum of disorders resulting from interactions with a variety of other organisms — bacteria, fungi, water molds, nematodes, insects and with biotic entities like viruses. With respect to these biotic causes of plant disease, plant pathologists describe what is called the ‘disease triangle’ where disease results from a combination of environmental conditions, host susceptibility, and the virulence of a disease-causing organism. Disease is a consequence of a combination of three factors, hence a triangle: a susceptible plant encountering a competent (i.e. virulent) pathogen under environmental conditions that favor the invasion and spread of the pathogen. A classic example of the disease triangle is late blight of potato, caused by the water mold Phytophthora. Potato is native to South America but was brought to Europe in the 17th century as a food crop. The disease organism, which also affects tomatoes, apparently originated in Mexico in the early 1800’s. The disease requires cool, moist conditions, a susceptible potato host and a virulent pathogen. The pathogen spread throughout North America in the early 19th century and made it to Europe probably as a result of importation of diseased potatoes to Belgium in 1845. It quickly spread throughout Europe, and in particular to Ireland, where potatoes were the primary food source and were grown extensively in monocultures. The cool moist conditions typical of Ireland were well-suited to the growth and reproduction of the pathogen. Moreover, the potatoes grown were genetically uniform and also highly susceptible to the blight. The result was devastating, over a million Irish died of starvation and another million emigrated, mostly to the United States. The severity of the blight varied over the next twenty years, primarily due fluctuations in environmental conditions. Note that crop failure not only eliminates food, it also eliminates ‘seed potatoes’ (potatoes saved to be planted the following year). Thus, even if favorable conditions might yield a relatively high crop (yield per acre), food shortages persist because fewer acres may have been planted. Late blight of potato continues to be a problem, with outbreaks tied to environmental conditions. There are partially resistant varieties but these are only temporary because the pathogen evolves to overcome the plant’s resistance.
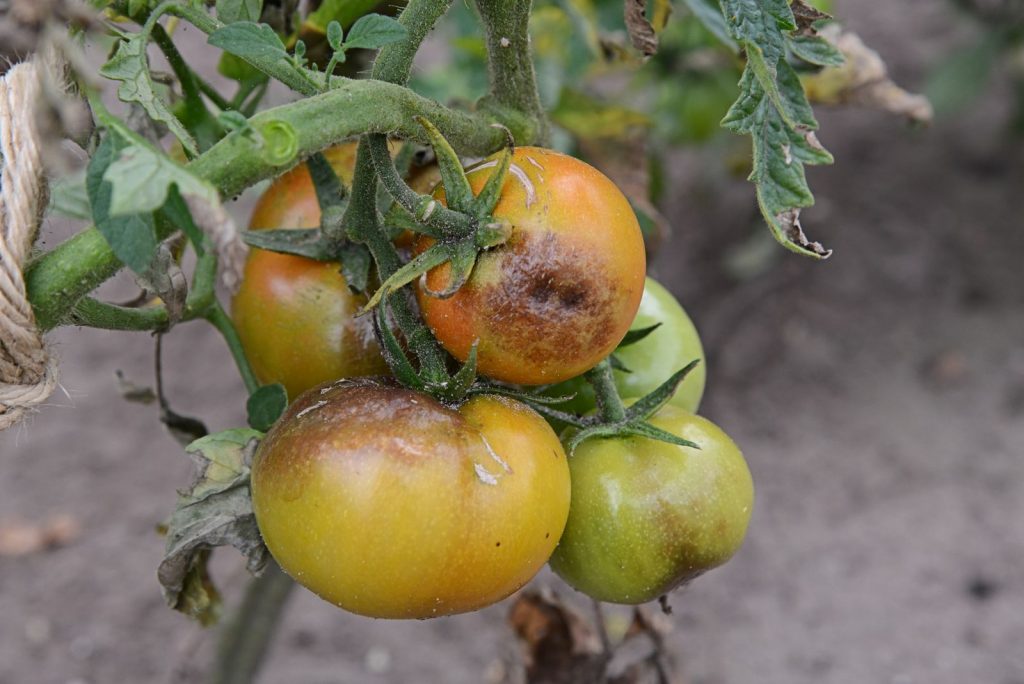
The pathogen is an obligate biotrophic parasite, meaning it can only survive on a living host (but it does eventually kill substantial parts or all of its host). It survives from year to year on potato tubers or potato plants left in the field. It spreads readily by spores (usually asexual) that require moisture to stay alive and are dispersed by wind and by raindrops. Pathogen growth and spread can be extremely fast if weather conditions are appropriate. The spores germinate quickly and enter susceptible plants through stomata, wounds, and directly through the cuticle. Once inside the leaf they produce hyphae that grow between cells and produce haustoria that penetrate the cell wall and interact with the host cell membrane and allow nutrients to pass to the fungus.
The disease requires a match between the host and the pathogen. P. infestans infects potato and also tomato, which is a close relative of potato. But many other close relatives are not suitable hosts. And there are other species of Phytophthora that affect other plant species but do not infect potatoes, e.g. P. quercina causes sudden oak death syndrome
Wheat rust—complex parasite life cycles
There are many different rust diseases that affect a variety of hosts, and some utilize two different hosts. All are host specific meaning that they can affect only a group of closely related plants: a variety, a species or several species from a single genus. The life cycle for wheat rust was introduced in Chapter 12 and a figure from that chapter is reproduced below. Note that five different types of spores are produced. They differ from each other by their ploidy level (diploid, dikaryon or haploid), what type of plant they are produced on, and what type of plant they can grow on (if any) and how they function. These are described below:
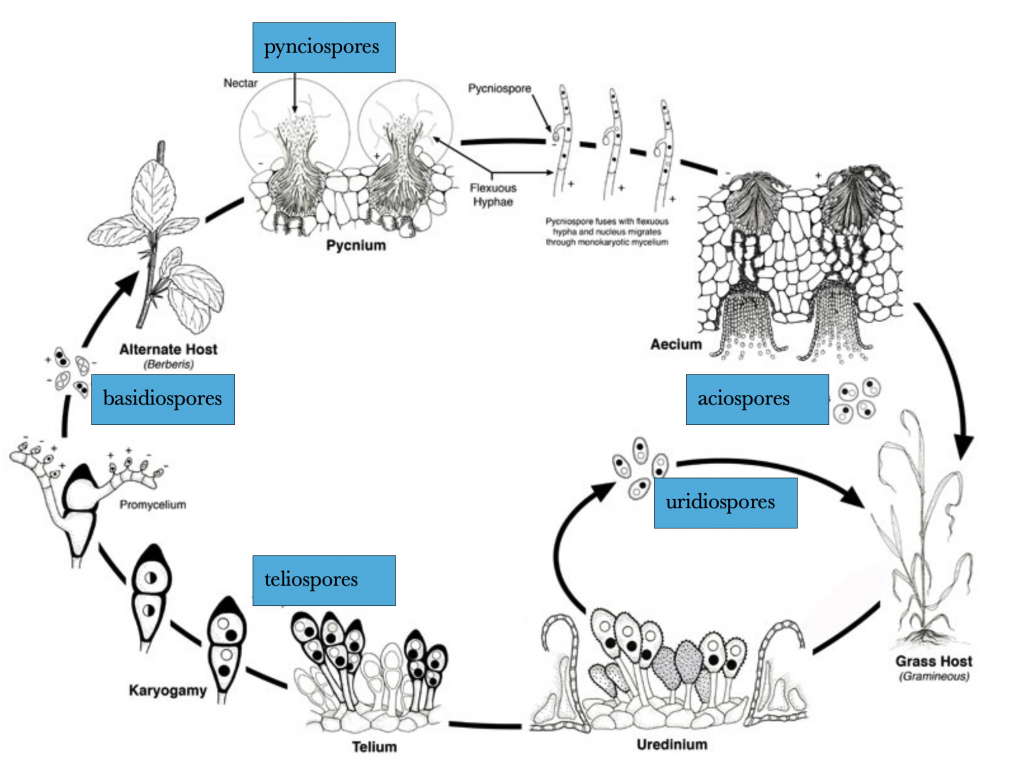
teliospores are dikaryon spores and the only stage of the life cycle that grows, albeit very temporarily, independent of a host. It also is the only stage that can overwinter.
basidiospores are haploid spores produced after the teliospore germinates and undergoes karyogamy to produce a diploid cell. This cell then undergoes meiosis to produce haploid basidiospores. These spores are dispersed in the air and only germinate and grow on barberry plants.
pynciospores are haploid spores produced from hyphae produced in a structure emerging from the upper surface of barberry leaves. Also in this structure are haploid receptive hyphae which can receive pynciospores and fuse with them (plasmogamy) to form dikaryon hyphae. These grow to the lower surface of the leaf and form a structure called an aecium that produces dikaryon aeciospores that are dispersed by the wind and infect wheat plants.
Mycelial dikaryotic growth from the aeciospores can cause significant damage to the host wheat plant and also produces urideospores, produced in and orange structures called uremia. The uridospores spread Puccinia graminis to other wheat plants, facilitating disease spread.
As the wheat plant starts to senesce, both from the pathogen and from its natural, monocarpic cycle, the dikaryotic hyphae in the wheat plant produce teliospores, spores that can survive the winter and thus completing the life cycle.
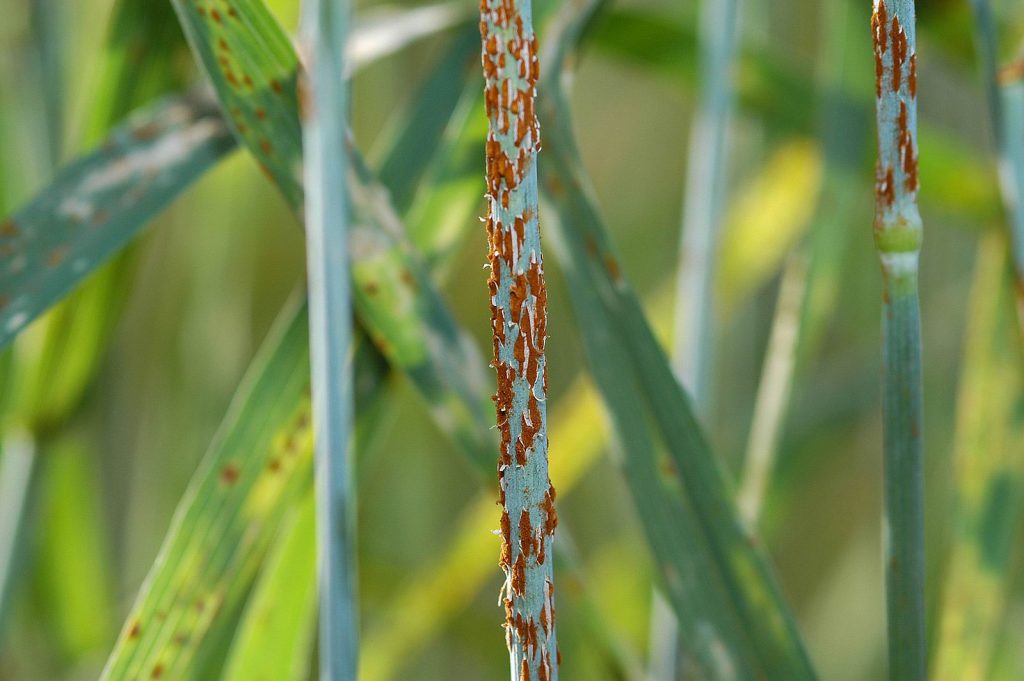
Because wheat is an extremely important crop and because the disease spreads rapidly and evolves quickly, wheat rust is probably the most significant agricultural disease. Resistance to the disease involves the genetics of both the fungus and the plant. Resistant plants have an ability to recognize invasion by the fungus and to respond to it. Recognition of the fungus is the result of the plant perceiving a chemical produced by the fungus. An avirulent fungus can become virulent by becoming unrecognizable, typically by not producing a specific chemical that that the avirulent (and thereby recognizable) fungi had been making. Hence virulence in the pathogen is typically recessive (inability to make something that the wild type does make). Plant resistance requires a dominant gene that gives the plant the ability to recognize and respond to the presence of the virus.
Corn smut—Mexican truffles
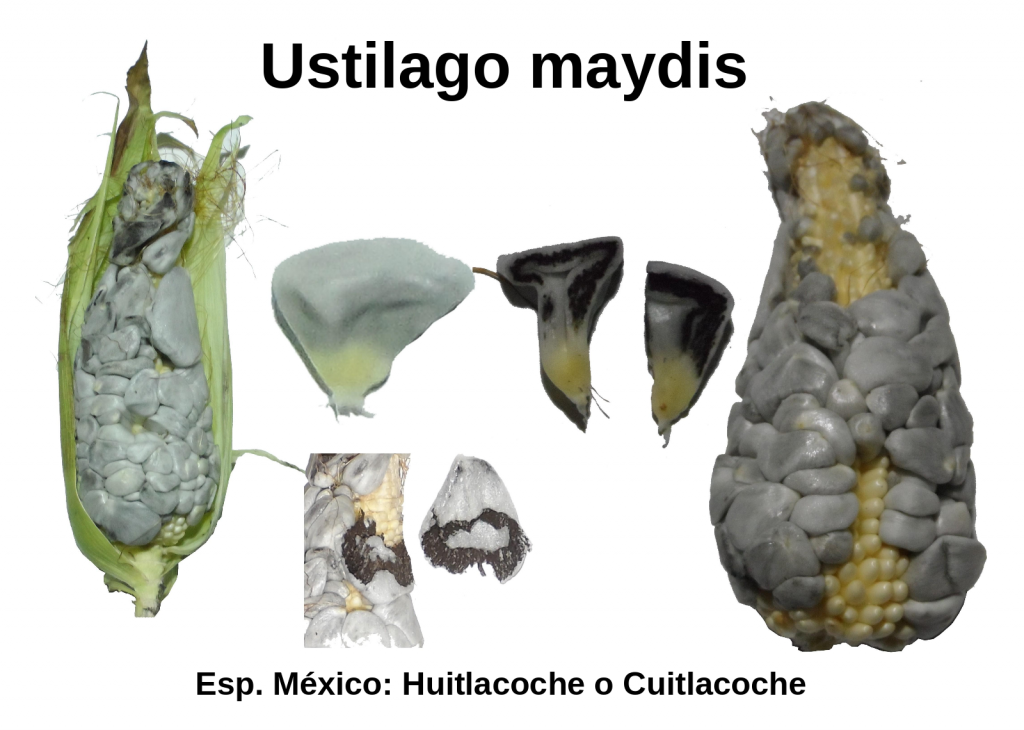
Corn smut produces large, distinctive galls that are edible and highly desirable in Mexico. In other parts of the world the disease is very unwelcome, substantially reducing crop yields. Like the rusts, smut diseases are basidiomycetes and like the rusts the fungus is generally found in the dikaryon state, with cells possessing two haploid nuclei. Basidiomycetes only produce only a single diploid cell that immediately undergoes meiosis to form haploid spores. These germinate and grow into haploid hyphae that, in smuts, can produce new cells by budding like yeast and like yeast can be grown on an artificial medium, meaning that this stage is NOT biotrophic but acts like a saprophyte. However, when haploid hyphae of two different mating types find each other and fuse and form a dikaryotic hyphae, it is now biotrophic, only able to survive on living cells of spe cific plants, in this case, corn and teosinte (a close relative of corn). Hence, to be a successful pathogen the haploid hyphae need to find each other on living corn (or teosinte) plants. Additionally, the dikaryon hyphae only invade active tissues, most commonly the flowers, where they induce abnormal growth, galls, with greatly enlarged cells surrounded by hyphae. They do not form typical haustoria but do form structures that allow the transfer of materials from the plant to the fungus. As the galls mature they change color from silky white to black and also change textures, becoming softer. Although the galls look ‘fungal’ (if such a thing is possible!) they are actually mostly plant tissue. Inside the galls some fungal cells undergo karyogamy (fusion of the two haploid nuclei) to form a diploid cell and complete the sexual cycle. Corn smut is the most significant smut disease but there are other smut fungi that infect other agricultural grasses (sugarcane, barley, oats) as well as smuts that attack wild grasses and sedges.
Rice blast disease—changing pathogen strategies
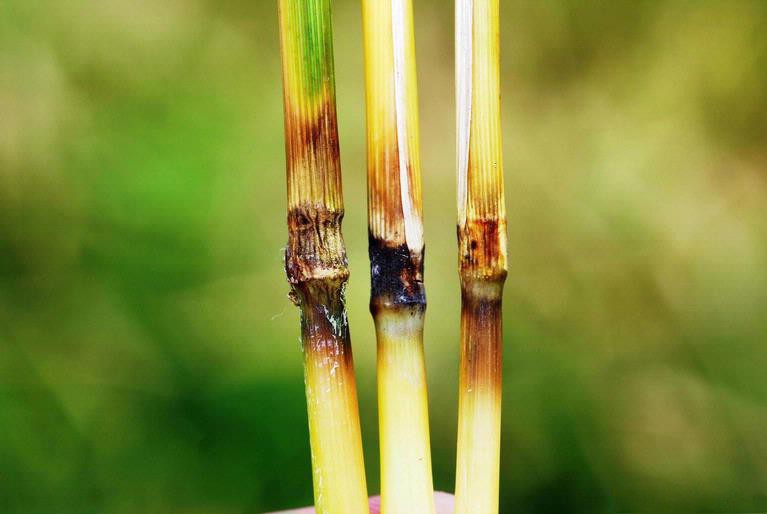
Rice blast is a devastating fungal disease that affects several cereals but is most significant in affecting rice. It is an ascomycete and the dikaryon stage is brief in time and extent. The pathogen is technically described as a hemibiotroph because initially it behaves like a biotroph, surviving on living plant cells, but eventually becomes a necrotroph, killing cells and obtaining nutrients from them. Haploid spores land on rice leaves, developing fruits, and other plant parts, and are able to penetrate the cuticle by generating substantial pressures hydrostatically within a specialized cell called an appressorium. Once inside the fungus is able to spread by entering individual plant cells through plasmodesmata. After a certain amount of time that depends upon the tissue that has been invaded, the fungus shifts to a necrotrophic lifestyle, killing the host cells.
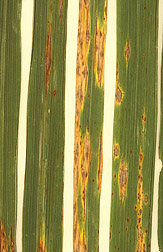
Dutch elm disease—overreacting can be deadly
Dutch elm disease is caused by an ascomycete fungus that has a symbiotic relationship with a bark beetle, a type of beetle that feeds on the vascular cambium and tissues (secondary xylem and phloem) that the vascular cambium produces. The fungus is transported from infected trees to new trees by the beetle and benefits not only by the transport but also by being placed inside the plant in the tissues that the fungus feeds on. The fungus feeds only on dying cells. but, unfortunately for the tree, its presence triggers the production of gums. Presumably such a response could help stop the spread of the fungus by making it more difficult to move. However, in elm trees the substances that are produced plug the vessels and trachieds of the host, making them unable to transport water up the plant. Like a number of human diseases, the damage of a pathogen stems mostly from the host response and less so from the actual activity of the pathogen. Dutch elm disease is one of many ‘wilt diseases’ caused by both fungi and bacteria that result in reduced water transport and therefore wilting. In most of these the blocking of xylem tissue is the result of the plant response to the pathogen.
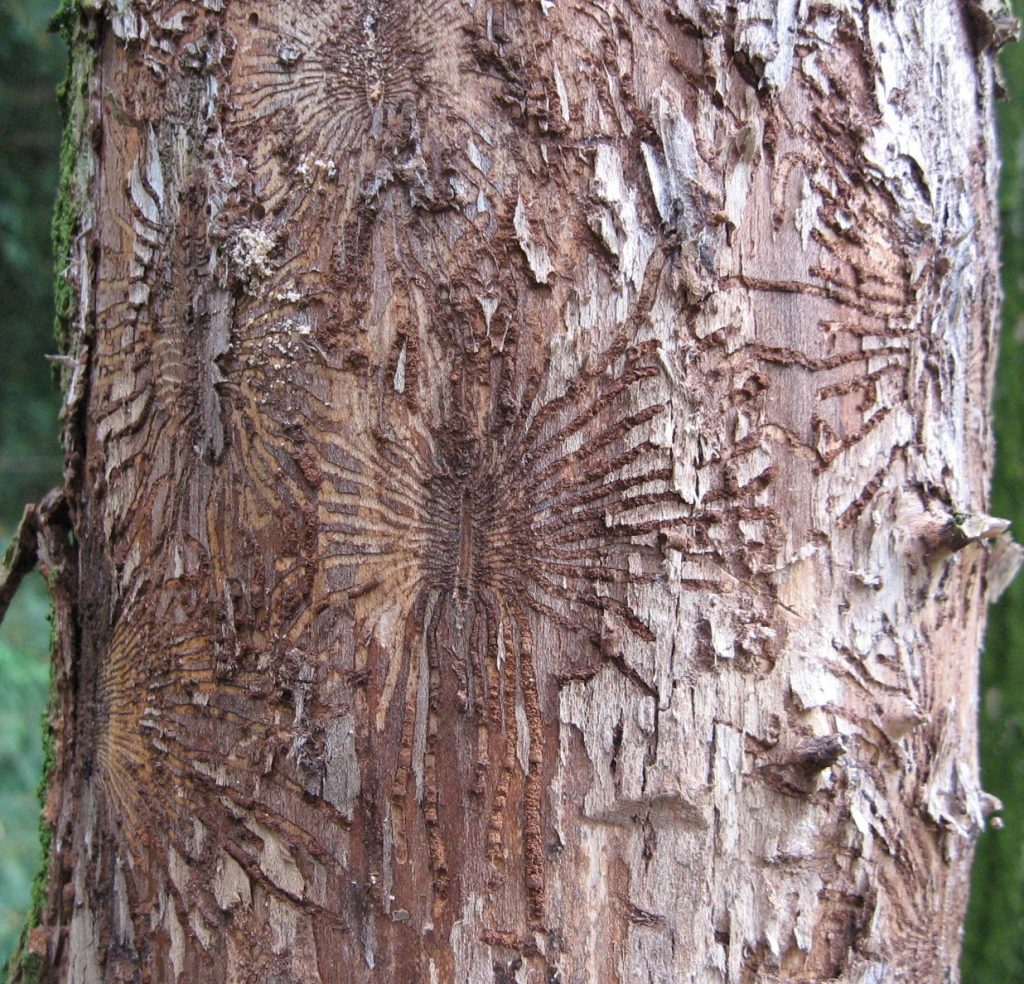
Fire blight and bacterial phytopathogens
The majority of plant pathogens are fungal, but some, like late blight of potato, are oomycetes and some, like the phytoplasmas of poinsettias are bacteria. While phytoplasmas are unusual because they are obligate intracellular parasites, most bacterial diseases do not enter into the cell but they do produce ‘effector’ molecules that they are able to transfer into living cells and effect specific results such as hormonal responses that bring about tumors, exudation of materials, or cell death. Most bacterial plant pathogens are not easily classified as biotroph/necrotroph although clearly phytoplasmas are biotrophs, as is the gall-forming Agrobacterium (see below). Fire blight is interesting in this respect. It is caused by Erwinia amylovora and affects members of the rose family, in particular apples and pears, sometimes with catastrophic results. The bacteria can live as a harmless epiphyte living on sugars exuded by the stigmas of flowers and the nectaries at the base of petals. From these locations E. amylovora can be spread by pollinators throughout a plant and throughout an entire orchard. At some point it is triggered to become a much less benign associate and it becomes necrogenic, killing host cells as a consequence of the effector molecules it produces.
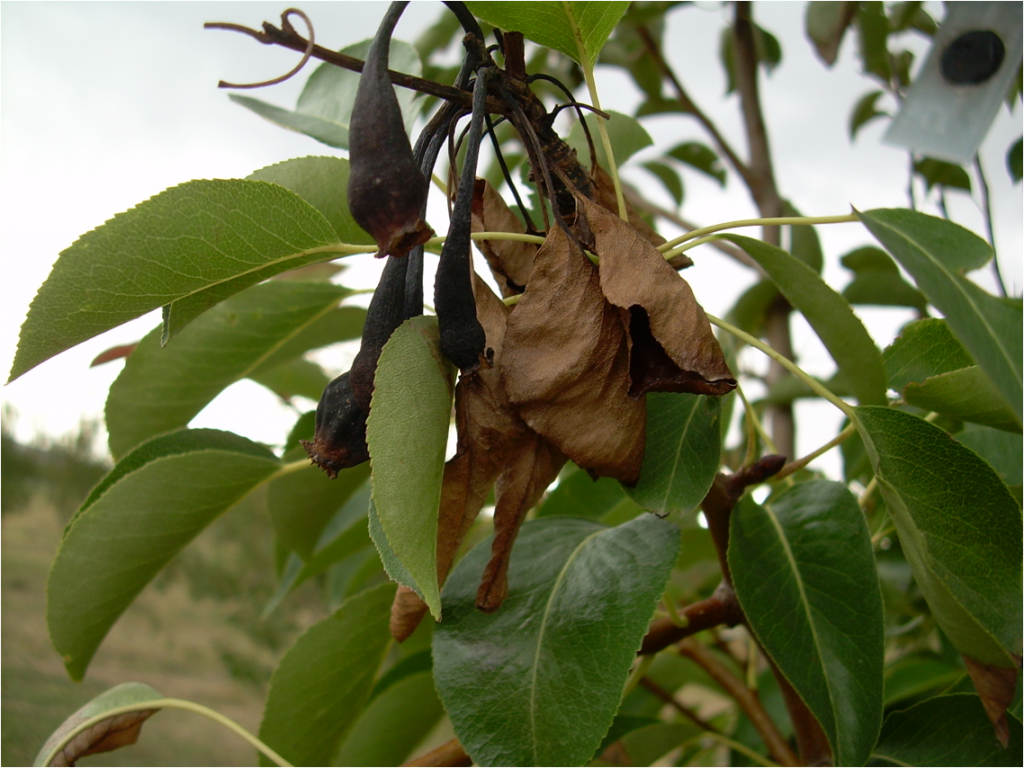
Crown gall disease—a disease used as a tool against disease
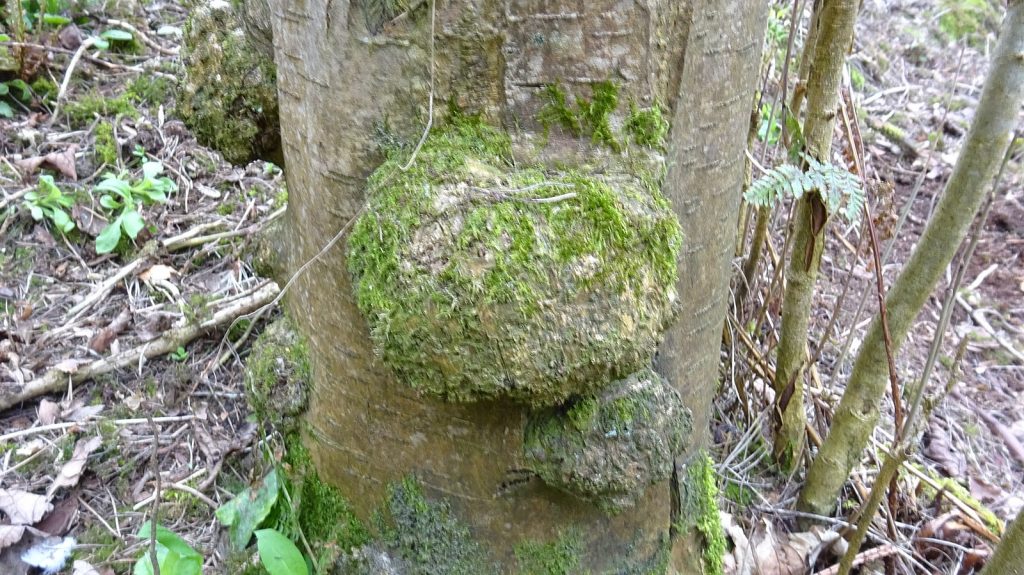
Many plant diseases alter the growth pattern of plants, often producing characteristic structures called galls. The abnormal growth that is manifested in a gall usually is a consequence of changes in the amounts of plant growth regulators, aka hormones. This can be the result of the pathogen producing these chemicals. In fact, one plant hormone, gibberellic acid, was first discovered as a result of it production by a fungal disease. But altered levels of plant growth substances can also be a consequence of the pathogen causing its host to produce more or less of a particular substance. The most common way this happens is that the pathogen alters gene expression in its host. Agrobacterium tumifaciens does this by actually altering the genes present in host cells via a process called transformation, a type of horizontal gene transfer. Among other genes that are transferred is one that codes for the production of the plant growth substance cytokinin which plays a role in the cell proliferation required for gall formation. The bacterium lives saprophytically in the soil but is able to recognize wounded plants when it contacts them and is transformed into a virulent form that makes its way into the plant. A. tumifaciens is a significant pathogen on several crop species, in particular perennial ones like walnut, apricot and plum. Agrobacterium tumifaciens is notable for its very broad range of hosts. This is one of the reasons why it has proved useful in the genetic engineering of plants where it is used to transfer specific genes from one organism to another (Chapter 31).
Tobacco mosaic virus, cauliflower mosaic virus, and ring spot disease of papaya
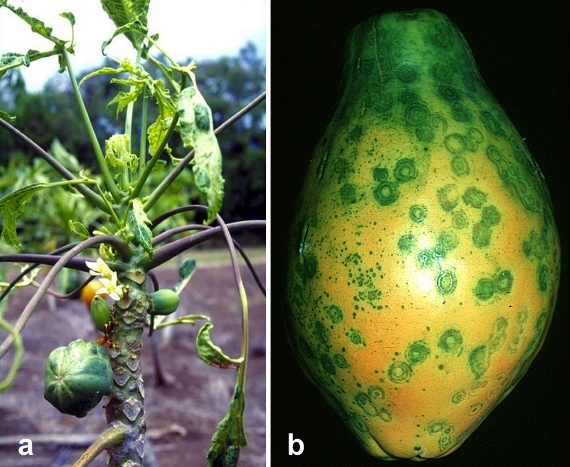
Plants are affected by a large number of viruses that can cause very significant crop losses. Tobacco mosaic virus (TMV) is not one of the most damaging viral pathogens, but it is notable both because of its role in the understanding of viruses and its use in bioengineering. TMV was the first virus to be isolated and purified and this allowed for the recognition that infectious agents need not be cellular in order for them to cause disease. Viruses are composed of both protein and genetic material (either DNA or RNA) and upon entry into a cell they insert genetic material that codes for the production of viral protein and genetic material. A critical part of the inserted viral genome is a region called a promoter that ‘promotes’ the expression of the genes downstream from it, thereby ensuring their expression. Genetic engineers have utilized this region of the genome of several viruses in order to insert desirable genes from one organism into another organism. In particular the cauliflower mosaic virus (CaMV) has proved to particular useful in producing ‘genetically modified’ organisms. One crop where the CaMV promoter was very useful was in the development of strains of papaya that are resistant to the ring spot disease of papaya, a disease that is caused by yet another virus that threatened to eliminate the papaya as a commercial crop.
Further Reading and Viewing
- “An Overview of Plant Defenses against Pathogens and Herbivores” by Brian C. Freeman and Gwyn A. Beattie.
- “Necrotrophic and biotrophic pathogens of plants” by David Moore, Geoffrey D. Robson, and Anthony P. J. Trinci.
- “Obligate biotrophy features unraveled by the genomic analysis of rust fungi” by Sébastien Duplessis et al.
- “Plants Rule – Biotrophic Versus Necrotrophic Fungi” by Helga George, Ph.D.
Media Attributions
- Bacterial Blight © Dean Malvick is licensed under a CC BY-NC-SA (Attribution NonCommercial ShareAlike) license
- Japanese Beetle Feeding on Peach Tree © Zech Smith is licensed under a CC BY-SA (Attribution ShareAlike) license
- Emerald Ash borer larvae © Pennsylvania Department of Conservation and Natural Resource is licensed under a CC BY (Attribution) license
- Oomycete © Emmanuel Boutet is licensed under a CC BY-SA (Attribution ShareAlike) license
- Poinsettia © André Karwath is licensed under a CC BY-SA (Attribution ShareAlike) license
- Phytophthora infestans © I.Sáček is licensed under a CC0 (Creative Commons Zero) license
- Tomatoes © Rasbak is licensed under a CC BY-SA (Attribution ShareAlike) license
- Wheat © Yue Jin is licensed under a Public Domain license
- Ustilago maydis © Sarumo74 is licensed under a CC BY-SA (Attribution ShareAlike) license
- Rice © Donald Groth is licensed under a Public Domain license
- Beetle galleries © Ronnie Nijboer is licensed under a CC0 (Creative Commons Zero) license
- Crown gall © Rosser1954 is licensed under a CC BY-SA (Attribution ShareAlike) license