Chapter 16: Reproduction: development and physiology
Reproductive/sexual behavior and the life of the organism
The act of reproduction is central to the concept of an organism and it requires transformations in the behavior and physiology of the organism, transformations from simple maintenance to something more. Even for the simplest of unicellular organisms, reproduction requires a shift in the ‘normal’ activities. The ‘cell cycle’ (Fig. 1) describes the pattern of activities that the cells go through in order to reproduce (i.e., undergo mitosis).
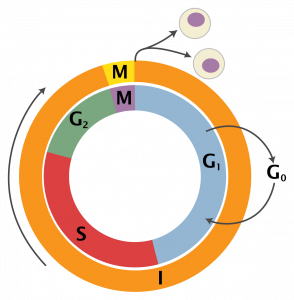
As the cell goes through this cycle its activities change. Changes in gene expression result in changes from ‘growth and synthesis’ activities (G1, S, G2 stages) to mitosis activities. The transformations are even more substantial if a unicellular organism’s reproduction involves sex. At some point in the sexual cycle meiosis has to be induced, requiring cells to drastically reorganize themselves in a manner that will halve the number of chromosomes in a very specific way. Additionally, cells with unique abilities, the ability to fuse with one another, need to be produced.
For multicellular organisms the required changes to bring about reproduction or to bring about the sexual process usually involve profound changes: new structures may appear and the and the behavior of the multicellular organism often shift s, sometimes in drastic ways, ways that may even end the life of that particular organism. This chapter considers some of the common patterns seen as organisms shift their physiology and structure in order to reproduce.
TOPICS
- Reproduct ive structures: production patterns in time and space
- Developmental control of sex and reproduction — general cues
- Patterns of reproduction in flowering plants
- Photoperiodism
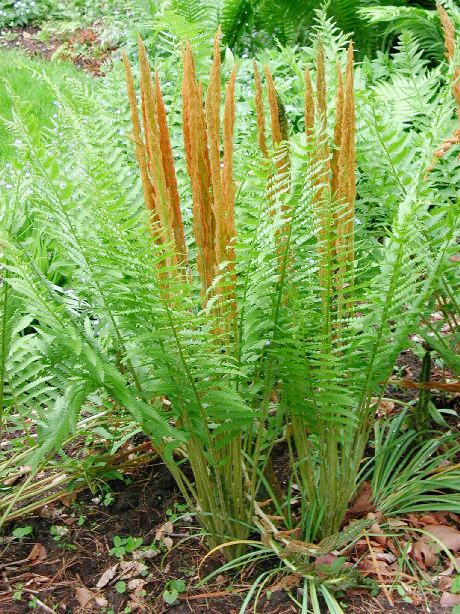
Reproductive structures: production patterns in time and space
For most organisms, the normal activities involve the acquisition of matter and energy and the act of reproduction shifts resources from structures that acquire matter and energy to those that are involved in reproduction. For a plasmodial slime mold the shift in structure and behavior is dramatic and involves the whole organism: the coenocytic film that has been spreading across the substrate, finding food, suddenly shifts to a completely different structure, one that is immobile and unable to feed but one that is able to produce propagules.
For other organisms, the processes of both sex and reproduction involve specific structures that are specialized for those purposes. These may be either continuously or periodically produced. For example, normal fungal growth produces hyphae that are able to explore the medium it is growing in and obtain materials needed for growth. To reproduce asexually, e.g., with conidia, a fungal filament grows in a different direction (usually upwards) and produces a series of small, easily detached cells that can be dispersed. Thus, the fungus has shifted some of its activities from its normal growth mode to reproduct ive mode. Another example would be dimorphic fronds in ferns (Fig. 2, see also sensitive fern) and horsetails; the plant makes one kind of leaf for reproduction and another type of leaf for photosynthesis–consequently its ability to photosynthesize is reduced as its ability to reproduce increases.
For some plants, sexual reproduction involves a dramatic reorganization comparable to the slime mold. Remember that a flower is a transformed branch and that branches are ‘new modules’, significant not only for their growth but also because of its potential to produce even more modules. The transition of that branch to a flower changes all this — it converts an indeterminate structure, capable of growing and producing more modules, into a determinate structure that produces a flower or flowers and then a fruit and then dies, eliminating that module, and removing its potential to produce any other modules. If all the shoots of a plant transition to flowering at the same time, then the plant as a whole becomes determinate and will die after fruiting. This is what happens in wheat (Fig. 3).
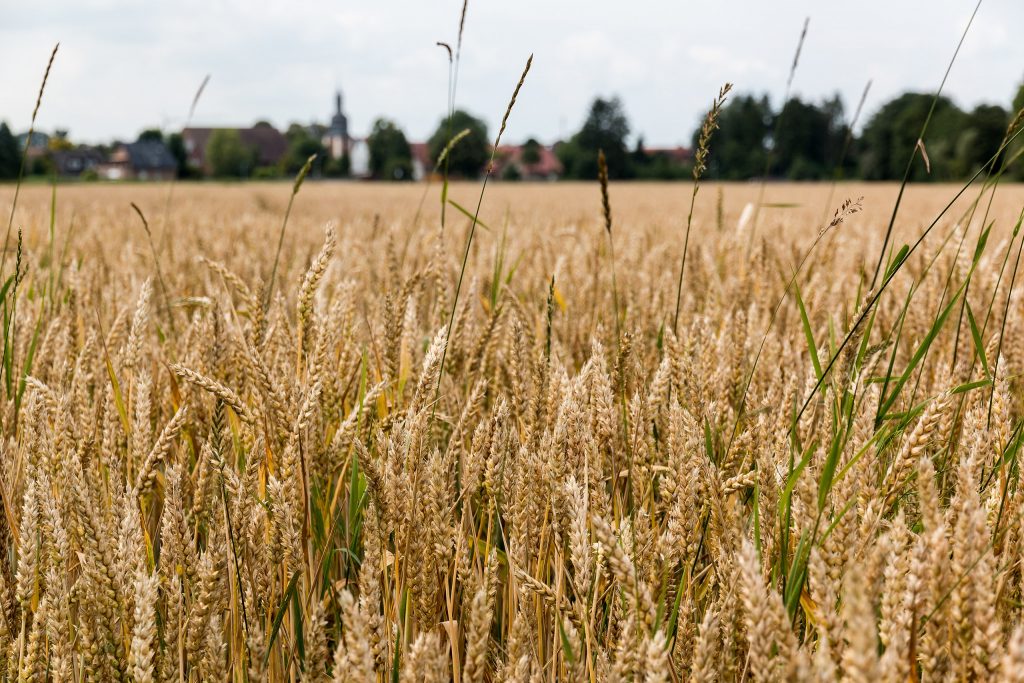
We can identify two ‘extremes’ in the pattern of allocation of resources to reproduction, one involves partitioning the organism in space and the other involves partitioning it in time. Some organisms, like cinnamon ferns, most algae and many fungi, simultaneously produce parts for reproduction and parts that conduct ‘normal’ activities. While the degree of partitioning may vary with time, the organism grows and reproduces more or less continuously. At the other extreme are organisms that spend part of their life growing and and then transform into a different type of organism in order to reproduce. Examples include butterflies, mayflies, carrot (Queen Anne’s lace) , burdock, beet and mullein, all of which produce a very short stem with multiple leaves and no branches in the first year of life. In the second year the stem elongates, branches form and flowers and fruits are produced. Most of the material stored from the first year of growth is directed to the developing seeds. In addition, the leaves are dismantled to provide additional resources. Consequently, the ability of the plant to photosynthesize disappears and the plant dies in the process of flowering and fruiting. In the first year the plant focuses on growth (acquisition of materials) , in the second, on reproducing.
Control of reproduction and sex
In most organisms the ability to reproduce is controlled by cues from the environment that are sensed and bring about changes in structure and allocation of resources. Although it is often assumed that reproduction (and hence population growth) is dictated by resources, there certainly are situations where resources are available but organisms do not utilize these resources to reproduce. Instead of being tied to resources, many organism s’ reproduction is tied to specific cues in the environment–they respond to these cues by reproducing. Stated another way, although adequate resources are necessary for reproduction, they are often not sufficient to bring about reproduction. Among other things this makes predicting population growth more difficult. This topic will be considered more when discussing the behavior of populations. Here we will discuss the particular cues that organisms utilize to trigger the reproductive process, sexual or asexual, and then consider the (primarily sexual) reproductive patterns found in flowering plants and some specific cues that control many plants’ reproductive patterns.
Cues to initiate reproduction and/or sex
Growth/development
For many unicellular organisms reproducing by mitosis, reproduction simply involves the achievement of a particular stage in cellular development. This is generally somehow tied to growth, i.e., the accumulation of enough materials that the cell can be partitioned in two. Growth is connected to time (‘cells have to reach a certain age to reproduce’) but only as mediated by material acquisition. Bacteria that divide every 20 minutes are not timing the intervals, there are processes taking place, some of them fundamentally tied to the acquisition of materials, that take 20 minutes to occur. If you change the temperature or change the availability of materials, bacteria will take a longer time to divide. Also, as noted above, organisms have the ability to change their rate of material acquisition, i.e., they control how fast they grow, and because of this, they can control their rate of reproduction.
Time
Although all eukaryotes appear to be able to keep time, very few have reproduction or sex cued specifically by time (i.e., have a stopwatch that is initiated and then reproduces at the end of a specific time). However, it is common, especially for plants that have been selected for cultivation, that an integration of time and temperature determines flowering. Plants need to grow to a certain stage in order to reproduce and this requires time and favorable temperatures. Many seed packets for home gardeners state something like ‘flowers in 60 days’— this is assuming a ‘normal’ temperature regime; if temperatures are cold, it might take 70 days. This idea of integrating time and temperature is discussed more in Chapter 26.
Nutrients
As discussed above, nutrition (i.e., the acquisition of resources required for growth) clearly can be a factor influencing growth and development, but sometimes nutrition plays a more specific role. Chlamydomonas sex is triggered by low nitrogen levels; fungal reproduction often requires a specific media formulation different from the medium that brings about growth; slime molds can be induced to sporulate (a phase of sexual reproduction) by specific nutrient regimes. The ability of some perennial plants to respond to flowering cues has been associated with carbon/nitrogen ratios in the plant that can be affected by both photosynthetic activity and nutrient availability.
Adverse/favorable conditions
Surprisingly, both good and bad conditions may trigger sex or reproduction, depending on the species. A number of organisms change their activities when conditions become unfavorable (e.g., high or low temperatures, drought, high or low pH, toxic levels of certain chemicals) and often the change involves reproduction and/or sex. For example, slime mold plasmodia (the multinucleate giant cells) are induced to form sporangia when the conditions are poor. A number of tree species are known to flower particularly well when they are about to die. These behaviors might be considered adaptive since it produces structures (seeds, spores) that are resistant to harsh conditions at a time when conditions are deteriorating. Such structures may also be beneficial because they also provide for dispersal and movement to new, and possibly more favorable, conditions. At the same time, a cessation or reduction in the production of propagules during adverse conditions can be justified because the resources are needed to keep the organism alive and not used on the ‘frills’ of reproduction and sex.
Cues that are useful in predicting upcoming conditions
Many species live in environments that vary seasonally and that have certain portions of the year that are much more favorable to activities related to reproduction/sex than other periods. The most obvious activity related to reproduction/sex is material acquisition but other factors might include: availability of dispersal agents (e.g., wind or perhaps specific pollinators or fruit eaters), lack of predators for offspring, etc. The most common cue that predicts upcoming conditions is photoperiod (the amount of light and darkness within a day). Photoperiodic control of flowering will be considered after describing the patterns of reproductive effort found in flowering plants.

Patterns of reproduction and sex in flowering plants
Angiosperms can be divided into two groups based on their flowering behavior: monocarpic plants, that flower a single time and die, and polycarpic plants, that flower multiple times. Monocarpic plants will die after flowering because all of their meristems have been converted from indeterminate vegetative shoots into determinate flo wering shoots. After flowering, the flowers develop into fruits and the plant dies both because there are no more vegetative shoots to produce more leaves and because the leaves that were already present have been ‘scavenged’ as a source of materials for the developing seeds and consequently have been destroyed. This was discussed above and is found in a number of crop species including corn, wheat, soybean (Fig. 3 and 4).
Many monocarpic plants are annuals, living for only a year. Whatever it takes to get the plant to flower occurs within a year of the germination of the seed. If a specific cue is involved, then within a year the plant develops a sensitivity to the cue and can respond. Note that some of the annual plants in northern (and southern) latitudes, especially those grown in gardens, may be annuals only because of the elimination of favorable conditions in the fall—they simply are killed by frost and cold and would live longer if the conditions were more favorable. Most of these plants are not native to this area; most of the annuals that are native to this area kill themselves off in the flowering process. In temperate North American habitats, most monocarpic annuals germinate in the spring and flower during the summer, but some, called winter annuals, germinate in the fall, overwinter and flower in the spring. Most of the wheat grown in the northern U.S. would be considered a winter annual, although in central and southern parts of the country the wheat grown is a regular annual and planted in the spring.
Monocarpic plants may also be biennials, sometimes defined as plants that live for two years but more accurately described as plants that live for two growing seasons. These plants are generally found in habitats that are seasonal, i.e., have part of the year favorable for growth and part unfavorable for growth, usually because of low temperatures, but occasionally because of lack of moisture. Biennials behave the way they do because during the first growing season they are not responsive to the cues that induce flowering, while in the second season they are. An example would be beet s, a species that needs a cold winter in order to be able to respond to the cues (photoperiod, see below) that trigger flowerin g. It does not flower the first growing season even though it receives the photoperiodic cue to flower but does flower the second year after being exposed to cold. Biennials are monocarpic and generally exhibit a substantial dimorphism between the first and second year, often having a very short stem and no branches the first year and elongating and branching stem the second year. As mentioned earlier, one might think of the form the first year being associated with acquiring resources and the form the second year being associated with reproduction.
Although uncommon, a few monocarpic plants are perennial, living for multiple years and then flowering once (Fig. 5). Examples include century plant (Agave), bamboo, and some gentian species. The case of bamboo is particularly significant to panda bears because they feed almost exclusively on the vegetative (i.e., non-flowering) bamboo plants which can grow for up to 70 or more years while forming an extensive clone. When the plant flowers, acres of bamboo, the product of 70+ years of growth, produces seeds (which pandas do not eat) and in the process the bamboo dies, leaving the panda with no source of food.
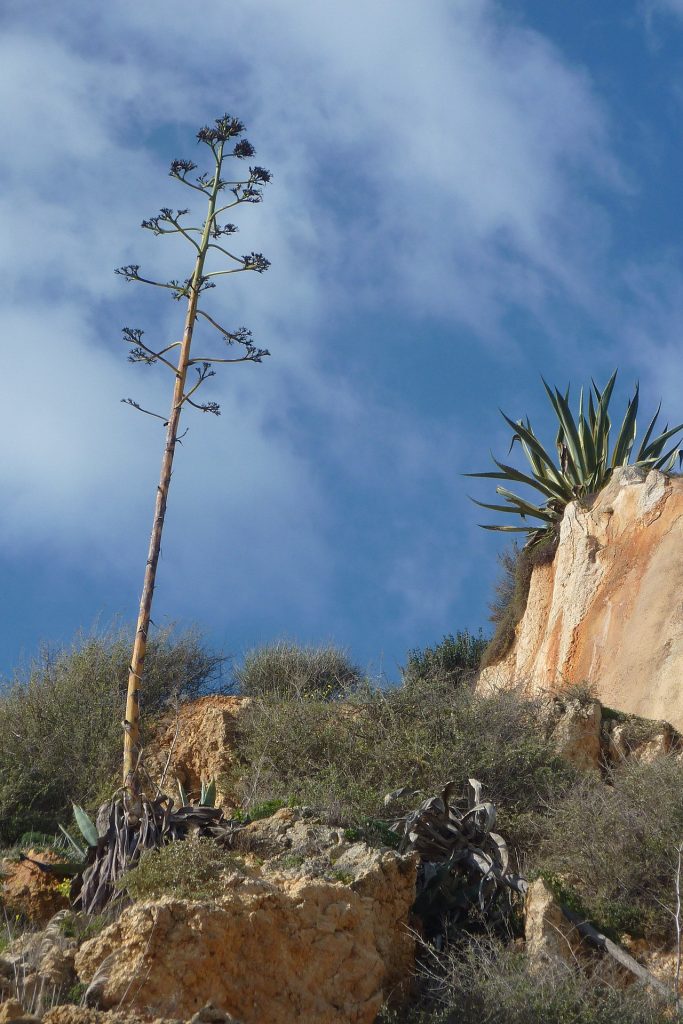
Polycarpic plants are perennial. They potentially live forever because only some modules are turned into flowering shoots on any particular year. Since all of the plant is exposed to the same set of cues, the different behaviors of different meristems, some producing flowers and some not, is the result of differing sensitivity to cues. A common pattern is that only stems that are one-year-old respond to the flowering cue (see coltsfoot). Thus, a plant will have two groups of shoots, shoots of the current year that do not respond, and shoots of the previous year that do respond, and therefore produce flowers and fruits and die. Hence, in the spring of the year, these plants are composed solely of the shoots that will become flowers. But before they flower, they produce branch shoots that will not become flowers until the next year. A version of this pattern is seen in many varieties of raspberry/blackberry. Although the plant is perennial, this is the result of underground stems. The vertical stems, called canes, are biennial, the first year growing vegetatively, the second year producing flowers and fruits and dying. A raspberry patch is perennial but the stems you see only live for two years, each cane behaving like a biennial.
Flowering plant cues for reproduction
While a few plants, especially plants selected for cultivation, require no specific environmental cue to induce flowering, they simply flower after the plant has grown sufficiently, the majority of plants require specific environmental cues to trigger flowering. However, for many plants, the cue alone is not sufficient to trigger flowering; the plant itself must be able to respond to the cue, i.e., the plant initially is not responsive and does not respond to particular cues. With time, the plant develops a sensitivity to the cue and can respond when the cue occurs. Thus, when we consider what makes a plant flower, we must consider the possibility that two processes are involved: one triggering responsiveness and a second triggering the flowering itself. A good example of this is the flowering of beet: in order to flower it must first be exposed to cool temperatures for a period of time; this develops its ability to respond to a specific cue that induces flowering, which is photoperiod, a particular combination of light and dark in a 24-hour period. Beet will not respond to photoperiod unless it has first been exposed to cool temperatures. Hence, it grows the first year without flowering, and only during the second summer, after a period of cool temperatures during the winter, will it be induced to flower.
Photoperiodism
A wide variety of organisms, including plants, animals, fungi and protists, respond to the photoperiod, relative amount of light and dark in a 24-hour period. For many organisms, including many plants, it is one of key determinants influencing reproduction (Fig. 6). Specifically for flowering plants, photoperiod often determines when a plant flowers, and photoperiod has probably been most extensively studied in this context. But it is important to appreciate that in addition to reproduction, photoperiod may affect a wide variety of organism features: physiology, structure, and behavior. Additionally, photoperiod’s mechanism of action is at the molecular level, i.e., by influencing which genes are being expressed.
Photoperiod is a significant cue for organisms living north or south of the equator because the photoperiod predicts upcoming conditions. The approaching winter can be sensed by the shortening photoperiods; an upcoming spring can be sensed by lengthening photoperiods.
Plants have turned out to be excellent organisms to study photoperiodism because, for some of them, a single day of a particular photoperiod can result in a measurable response. In contrast, for some organisms, and indeed for most plants, a response is only apparent after prolonged exposure to particular photoperiods.
Early studies established the fact that it is the night period that is critical. An interruption of the dark period can change a response while an interruption of the light period does not alter behavior. The ability to respond to photoperiod requires two abilities: the ability to sense light vs. dark, i.e., a photosensor, and the ability to keep time. In all organisms studied, the timing mechanism appears to be associated with an internal 24-hour rhythmicity, something described as the circadian clock because the rhythms in behavior have a periodicity of around (circa = around) 24 hours. A circadian rhythm is probably found in all eukaryotic organisms, certainly it seems to be a feature of most eukaryotic organisms where it has been looked for.
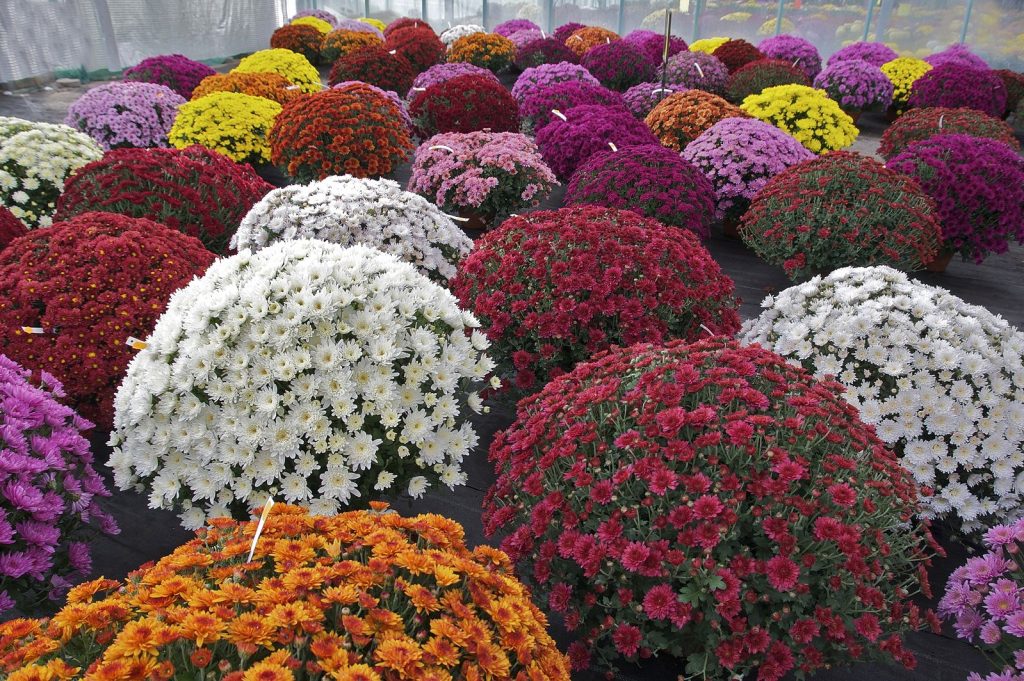
The actual photoperiodic response is the result of a particular pattern of light and dark that is imposed on an organism’s internal 24-hour rhythm. As a result, the behavior observed after giving an organism 12 hours of light and 12 hours of dark depends upon what portion of its 24-hour cycle that the 12 hours of dark is applied. The timing mechanism is not a stopwatch that times the dark period, what is critical is how periods of light/dark interact with internal rhythms that have a 24-hour periodicity. Stated differently, there is a periodicity in plants response to darkness.
The most commonly encountered photoperiodic responses involve reproduction but more generally photoperiod can be an organizing factor determining the patterns of growth and development that an organism exhibits. Some of these are listed below:
In flowering plants:
- flower production
- temperature tolerance
- production of vegetative buds
Fig. 7 Potatoes form when the tips of short underground stems enlarge and store carbohydrates. This transformation is a photoperiodic response, triggered by shorter days. - activation of buds
- activation of lateral buds
- formation of tubers (Fig. 7)
- seed gemination
In non-flowering seed plants:
- production of cones
- formation and activation of buds
In dinoflagellates:
- cyst formation
In both red and brown algae:
- pattern of growth and formation of reproductive structures
In animals:
- development of ovary and testes in birds and other animals and consequent changes in behavior
For flowering plants, the photoperiodic flowering response is generally put into one of three categories:
- long-day plants, which flower only if daylengths (periods of light) are longer than some critical value.
- short-day plants, which flower only if daylengths (periods of light) are shorter than some critical value.
- day neutral plants whose flowering is not obviously tied to photoperiod.

Short-day plants (black line) need night periods longer than some critical value in order to flower (Fig 8). Long-day plants (red line) need night lengths shorter than some critical value in order to flower. The actual critical daylength (i.e., the vertical part of the line) may shift to the right or left depending upon the particular species (or variety within a species). Note that neither of the two plants illustrated here would be flowering with night lengths between 15 and 12 hours. Similarly, if the red line were shifted enough to the right, or the black line enough to the left, one would have situations where both long-day and short-day plants would flower at the same photoperiod.
Although the names refer to periods of light in a 24-hour period, it is actually the night period that is critical. Hence long-day plants might better be called short-night plants and short-day plants might better be called long-night plants (but they aren’t!!). Another confusing factor to appreciate is that although we might consider that any day with more than 12 hours of light is a ‘long-day’ (and ‘short-night’), what is critical is the actual length compared to the critical value. Hence, one can have both long-day and short-day plants flowering under the same photoperiod: a photoperiod of 10 hours light and 14 hours dark would trigger flowering in a long-day plant with a critical value of 9 hours of light and also a short-day plant with a critical value of 11 hours of light.
While plant responses fall into these three general types, the actual responses are often complicated by two factors. One is that a plant response to a particular treatment may not necessarily ‘all or nothing’ (described as a ‘qualitative response’), meaning that the plant will not flower unless it receives the appropriate stimulus. For many plants the response to an appropriate stimulus is ‘quantitative’ meaning that the plant flowers more quickly or with more flowers if receiving a particular stimulus (the transition lines on the graph above may not be vertical but instead have a slope). A second complicating factor is that photoperiodic sensitivity may involve multiple sequential signals, including ones that do not involve photoperiod. For example, some plants will only respond to long-days after short-days or respond to long-days only after a period of time under cool temperature conditions.
In plants there appears to be two pigments, phytochrome and cryptochrome, that can interact with circadian rhythmic phenomena and produce photoperiodic responses. While both of these pigments interface with several different physiological processes, the flowering response appears to be the result of changes in gene expression resulting from signal transduction systems that influence protein/DNA interactions. Specifically, the appropriate photoperiodic stimulus appears to result in production of specific mRNA molecules that are important in transforming vegetative shoot apical meristems into floral meristems.
Further Reading and Viewing
- “The Molecular Basis of Diversity in the Photoperiodic Flowering Responses of Arabidopsis and Rice” by Ryosuke Hayama and George Coupland. A good summary of the molecular basis for photoperiodic phenomena.
Media Attributions
- The cell cycle © Zephyris is licensed under a CC BY-SA (Attribution ShareAlike) license
- Cinnamon fern © Robbin Moran is licensed under a All Rights Reserved license
- Wheat plants © Dietmar Rabich is licensed under a CC BY-SA (Attribution ShareAlike) license
- Agave © Muffinn is licensed under a CC BY (Attribution) license
- Chrysanthemum flowers © Jebulon is licensed under a Public Domain license
- Potatoes © BASFPlantScience is licensed under a CC BY (Attribution) license