Chapter 11: Reproduction and sex
Reproduction is an essential component of organisms—organisms are ‘living things’ that can replicate themselves, i.e., reproduce. While some other ‘living things’ (e.g., cells, some organelles) can reproduce, many forms of life cannot (e.g., tissues, organs (usually) , many communities). We have defined organisms as biological entities that are discrete in time, i.e., they have a beginning and ending; organisms originate as the result of a reproduction event carried out by some already existing organism(s). Although sex is often associated with reproduction, it is a distinct phenomenon. And many organisms, especially non-animal organisms, usually reproduce in ways that do not involve sex and some have no sexual process at all.
TOPICS
- Reproduction — single celled organisms
- Reproduction —multi-cellular organisms
- Reproduction and dispersal in space
- Sex
- Examples from the green, red and brown algae
- Chlamydomonas
- Oedogonium
- Fucus
- Saprolegnia
- Lamanaria
- Ulva
- Porphyra
- Polysiphonia
Reproduction—single celled organisms
For single celled organisms, reproduction is a familiar and conceptually simple process (although the cellular details are far from simple!)—cell division. The original cell is partitioned by the synthesis and deposition of a new boundary (Fig. 1). For all cells, the boundary includes two plasma membranes (one for each cell) and for organisms with a cell wall the new boundary also has wall material between the two membranes. Note that for cell division to be a means of reproduction the daughter cell needs to detach from its parent cell either immediately following cell division or at some point in the future. Otherwise the new cell is simply increasing the size of the existing organism.
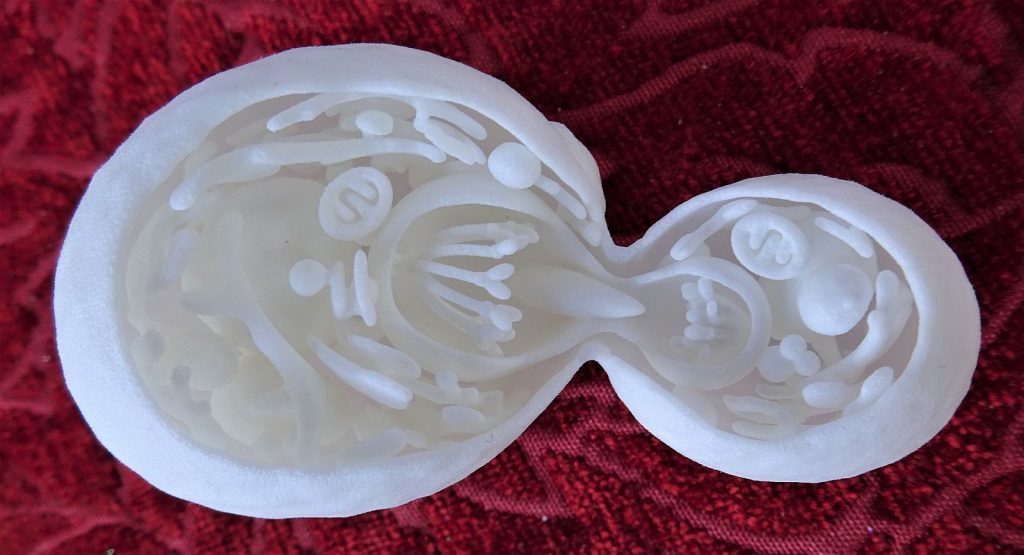
The original cell need not be divided equally, but both cells must be provisioned with whatever is required for the cell to maintain itself, in particular it needs genetic information in the form of DNA. Consequently, before the cell divides it needs to replicate its DNA and give each daughter cell a copy. For eukaryotic organisms, the DNA is packaged in units, chromosomes, that replicate before division, with one copy going to each daughter cell, i.e. the process of mitosis. Also present in eukaryotic cells are organelles (e.g. mitochondria) that need to replicate before cell division, with at least one ending up in each daughter cell. For photosynthetic organisms the same would be true for plastids that develop into chloroplasts. Remember that most biologists consider mitochondria and plastids to be remnants of unicellular organisms and their ability to divide is support for this idea.
Reproduction has to involve growth at some point but, as discussed in Chapter 7, growth might come after cell division (a cell is cut in half and then each half grows to full size) or before (a cell grows to twice its normal size and divides); ultimately reproduction requires that the organism acquire material, but this does not need to be a direct part of reproduction.
Although basically simple in outline, cell division, and the reproduction that it brings about in unicellular organisms, involves a myriad of details, some of which are unique to particular groups and are used to unite (classify) organisms.
Reproduction—multi-cellular organisms
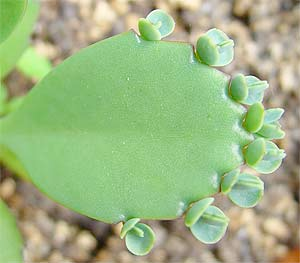
Reproduction in multicellular organisms involves another level of complexity because it requires the coordinated production of multiple cell types positioned in time and space in ways that achieve a functional organism (i.e., a developmental process, see Chapter 7). The information to direct this development is present in the DNA, and since most, or all, of the cells of an organism possess the same DNA, any of these potentially could develop into a new, multicellular organism. However, most cells are unable to express the required information to direct the production of a new organism and thus are NOT able to proliferate and develop a new organism. The developmental potential that can transform a single cell into a multicellular organism generally only finds expression under very limited circumstances. For most familiar organisms (mammals) this potential is only revealed in a single cell, the zygote (defined and discussed below). In these organisms, in spite of the fact that all cells have the same genetic information, only the zygote uses it to develop into a new organism as it undergoes embryogenesis and transforms into an embryo. However, in many of the organisms studied in this course, this developmental potential is encountered more frequently, and cells that aren’t zygotes can develop into new multicellular organisms in a process described as somatic embryogenesis. This may occur spontaneously or may be induced to occur. Bryophyllum, or mother plant (Fig. 2), nicely illustrates this. On the margins of its leaves new plants develop. This is possible because certain leaf cells behave like a zygote, using their potential to develop into a new organism (at least they will be new organisms when they detach from the parent plant). Another plant exhibiting the same ability is bulb-bearing fern (Fig. 3) which also produces structures on its leaves that detach and grow into new plants.
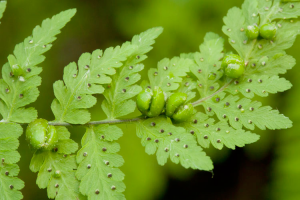
New multicellular organisms do not necessarily have to originate from the proliferation of a single cell. Reproduction can be achieved by the cleaving of an existing organism into two or more parts, as long as the resultant pieces of an organism are capable of regenerating the missing parts, or if the organism is so simple that it doesn’t have parts, e.g., a filamentous algae or a fungus. Such a pattern of reproduction is very common for many of the organisms covered in this book. Since a new organism can be produced by breaking a piece off an existing organism, a means of enhancing reproduction would be to have pieces that easily break off. This happens in Bryophyllum and in bulb-bearing ferns and in many other organisms as well. Both mosses and liverworts commonly produce ‘splash cups’, cup shaped organs (Fig. 4) that have at their bottom clusters of cells packaged into disk-shaped gemmae (singular gemma) that are easily dislodged and can be thrown out of the cup by a water droplet whose force is focused by the shape of the cup.
Reproduction and dispersal in space
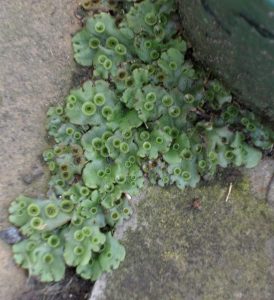
The phenomenon of splash cups points out that reproduction isn’t just about making new organisms, it is also about moving organisms around, i.e., dispersal. For many of the organisms dispersal may be the most significant aspect of reproduction, far more important than the making of a new individual. For familiar (determinate) organisms like mammals, reproduction is the only way to be perpetuated through time. In contrast, organisms that are indeterminate can potentially live forever, so continuation through time may not be a significant consequence of reproduction, but dispersal often is. Environments are dynamic both in their physical conditions and their biotic conditions, thus the fact that an organism can survive at a particular time in a particular place does not ensure that it will be able to do so in the future. Particularly for the organisms covered in this book, most of whom are immobile, it is often the case that reproduction is less about making new individuals and more about getting to new areas. ‘Pando’, the clone of aspen growing in Utah (see Populus), apparently has perpetuated itself for 80,000 years just by growing. Although in the past 80,000 years it has spread to new areas, it is not moving around very quickly. And, significantly, it is growing in an area where seedling establishment is impossible (i.e., conditions are less hospitable now than they used to be).
Getting to new areas involves the production of propagules, a unit that not only makes new organisms (reproduction), it also can put them in a new place. A fungus that produces a million spores may seem impressive but the consequences in its immediate area may be trivial. Similarly, a pine tree that produces thousands of seeds may be doing nothing to its functional population size at that site and at that time; the more significant effect of producing propagules is enhancing the possibility of establishing a population at some distant site or this site at some time in the future. Common propagules are spores and seeds but certainly the ‘bulbs’ of bulb-bearing ferns and the gemmae of mosses and liverworts should be considered propagules as well.
Probably the most significant feature that most propagules possess that enhances their dispersal ability is the fact that they are generally inactive, ‘dormant’. A general feature of cells and organisms is that the less active they are the less sensitive they are to a variety of ‘insults’, in particular desiccation and adverse temperatures. Cells and tissues that are in an inactive state, e.g., most spores, seeds, tree buds in the winter, can withstand conditions that active tissues cannot. The changes that accompany inactivity include changes in membrane and protein structure. An indicator of inactivity is a low metabolic (respiratory) rate associated with a general lack of any cellular activity such as protein synthesis or cytoplasmic streaming. Because of their inactivity, many spores can survive prolonged periods without the basic requirements that most organism require: moisture, matter and energy availability, temperatures within a particular range. This aids in dispersal simply because the propagule can travel longer distances while still being viable. Becoming dormant is a physiological process that may be triggered by specific environmental conditions or may simply be an aspect of a developmental pattern, e.g., a cell is programmed to become inactive soon after being created. What triggers the resumption of activity in a propagule varies from the return to ‘favorable conditions’, e.g., warmth and moisture, to more specific environmental cues, e.g., photoperiod. Some of these are discussed in Chapter 16.
The two common propagules found in the organisms covered here are spores and seeds. Seeds are only found in some plants and will be discussed in depth later. For now, simply appreciate that seeds function both in dispersal and reproduction. Spores are found in almost all of the organisms covered here (Bacteria, Archaea, Fungi, most protists, all plants (including those that have seeds). Their function varies considerably, from being a structure primarily associated with perpetuation in time (endospores of bacteria, akinites of cyanobacteria, zygospores of bread molds) to functioning primarily for reproduction and dispersal (zoospores of water molds and Oedegonium) or primarily for sex (considered later in this chapter and in Chapter 14).
Many organisms produce multiple types of spores which may differ in their structure and function, e.g., degree of dormancy, specific requirements required for resumption of growth (see bread molds, Oedogonium). A common type of spore is called a zoospore, a flagellated spore found in many of the green algae, most of the water molds, many brown algae, and many of the chytrids (the only fungi that possess flagellated cells). Zoospores reflect the costs and benefits tied to mobility: their flagella provide them with the mobility that unflagellated cells lack, but flagella require metabolic activity and consequently zoospores have limited lifespans and this limits their mobility and dispersal ability. Another trade-off relates to size. Spores are single cells and generally very small. This enhances mobility by allowing for dispersal by wind. However, any spore benefits by storing material that will be utilized in establishing the new growth following spore germination. The more material saved the heavier the spore is and the more limited its dispersal. These same considerations are significant to seeds, which are multicellular propagules considered in a Chapter 14.
Although reproduction is obviously of significance to the organism(s) that are produced, one final general point about reproduction concerns its impact on the ‘parent’ organism. Because reproduction utilizes material and energy that might otherwise be used to perpetuate the life of the parent organism, reproduction generally diminishes the likelihood that the parent will be perpetuated through time. The magnitude of this detrimental effect varies from highly significant, when reproduction insures the death of the parent (salmon, wheat plants) to extremely trivial, when reproduction has virtually no effect on the survival of the parent. Because of this impact on the reproducing organisms, reproductive effort is evolutionarily modified and often controlled by specific environmental cues (Chapter 16). Besides the impact at the individual level, reproduction also has potential consequences at the population/species level, increasing the population size and perpetuating the species through time. This effect is strongly dependent upon on other conditions.
Sex
Most students equate sex and reproduction, but they really are two separate processes that happen to be combined in the organisms that we are most familiar with. Reproduction is about making new organisms; sex is about mixing the genetic information of two organisms. Bacteria and Archaea exchange genetic information by several different processes (conjugation, transformation, transduction) but none of these are considered to be sex. Sex is defined as a particular type of genetic exchange that can only happen in organisms with chromosomes (eukaryotic organisms). Sex requires the fusion of two cells (syngamy), producing a cell with twice the number of chromosomes as either of the parent cells. Generally, both of the fusing cells have one copy of each chromosome and are described as being haploid, while the fused cell has two copies of each chromosome and is described as being diploid. Sex also requires a mechanism that can produce haploid cells from diploid cells. This process is meiosis, unfortunately often described as a ‘type of cell division,’ but is more aptly described as a process involving two cell divisions that produces haploid cells from diploid cells. Keep in mind that the haploid cells that are produced don’t simply separate the chromosomes of a diploid cell into two groups, the two groups each have one copy of each ‘type of chromosome’. Consider Arabidopsis (‘Mouse-ear cress’), the most studied plant in the world. If one looks at the chromosomes of a diploid cell, one sees ten chromosomes. But closer examination of the chromosomes reveals that there are actually five distinct types of chromosomes present, and their are two chromosomes of each type. (A chromosome can be recognized by its size and shape. And genetic analysis reveals that they are also distinct in the genes that they possess.) In Arabidopsis, meiosis produces cells with five chromosomes not ten, moreover, it produces cells that have one of each type of chromosome, i.e., a complete set.
Sex is a process that allows genetic material (genes) from two different organisms to be mixed. It almost always involves producing new individuals (reproduction). However, the unicellular organism Paramecium demonstrates that sex can happen with no reproduction: two cells, each with a diploid nucleus join temporarily. The diploid nucleus of each cell undergoes meiosis to form four haploid nuclei, three of these disintegrate and the remaining one divides mitotically to produce two haploid nuclei in each of the joined cells. Each cell sends one of these two nuclei to the other cell so that both cells have two nuclei, its ‘original’ one and one that came from its partner. Finally, in each cell, the two nuclei fuse to form a diploid nucleus, the original condition. Thus, the cells have undergone the sexual cycle but have not reproduced: there were two cells at the beginning and there are two cells at the end. Although sex and reproduction are different processes, they often (especially in familiar organisms) occur simultaneously.
Specifically, sex requires:
- Fusion of two cells and the subsequent fusion of the two nuclei in a process called syngamy, combining the genetic information of each. A ssuming that the original cells had one copy of each gene (i.e., the cells were haploid), the product of fusion will have two copies of each gene, i.e., will be diploid. Only special cells have the ability to fuse with one another and these cells are called gametes.
- A process (meiosis) that starts with a diploid cell and produces haploid cells, each with one copy of each chromosome. This process is feasible in eukaryotes because they have genes that are packaged into structures (chromosomes) that can be sorted and moved. Describing a cell as ‘having two copies of each gene’ is the same as saying that the cell has ‘two copies of each chromosome’(in fact it is more accurate since it is commonly the case that genes often get replicated, i.e. a haploid cell commonly has multiple copies of a gene, sometimes all copies are on one chromosom e, other times it has copies of a gene on several chromosomes.)
Most students consider sex to be related to the fusion process, but it is important to appreciate that meiosis is also an essential part. The essential components of sex (syngamy and meiosis) are sometimes distantly separated in time and may be separated between organisms. We will study a number of situations where two types of organisms are produced, both associated with the same species; one organism develops from a diploid cell (zygote) produced by syngamy and is diploid; the other develops from a haploid cell (spore) and is haploid. However, there are other organisms where the cell created by syngamy immediately undergoes meiosis, i.e., both steps of sex, syngamy and meiosis, occur in the same cell. As we will see there are lots of variations on the basic sexual cycle.
Sex is not universal. Many organisms, including some very successful groups (Archaea and Bacteria, the endomycorhizal forming Glomeromycota, most dinoflagellates, many fungi) have no sexual process. While sex is generally considered to be significant to the process of evolution because it promotes the variation that natural selection can act upon, it is important to realize that variation and evolution can occur without sex and that the success of a group of organisms at one point in time, and through time, is possible even if that group has no sex.
For multicellular or colonial organisms, reproduction, unless occurring by fragmentation of an already existing colony/organism, requires a cell that will proliferate and form the colony/organism. In the familiar case of humans, that cell is the zygote. But in many organisms it is a haploid cell produced by meiosis, generally called a spore, that has the developmental potential to proliferate and form a multicellular organism. And for all plants and many of the macroalgae, multicellular/colony development can proceed both from a zygote and from a haploid spore produced by meiosis. Such organisms will have both a haploid stage, derived from the spore, and a diploid stage, derived from the zygote.
The rest of this chapter will illustrate several examples of sex and reproduction, showing a diversity of patterns from several different groups. It is important to realize that for many of the larger groups (generally phyla) that we study, in particular for the macroalgae (green, red and brown algae), sex and reproduction are NOT consistent across the group, i.e. there is no single, standard pattern of sex and reproduction. In separate chapters we will consider groups that do show some consistency: with several of the fungal phyla (Chapter 12), with non-seed plants (Chapter 13), with seed plants (Chapters 14 and 15).
Chlamydomonas
Chlamydomonas is a unicellular green alga that primarily reproduces asexually (left side of diagram below), i.e,. most new cells are not the product of a sexual process. On the right side of the diagram is shown the sexual process which is trivial in terms of reproductive effort but significant for the variation that it creates and also because the thick-walled ‘zygospore’ (so named because it develops from a zygote) is able to withstand hostile conditions (dispersal in time). The sexual process is ‘triggered’ by conditions that make normally asexual cells behave like gametes, with the ability to fuse with other cells. The only diploid cell is the zygospore.
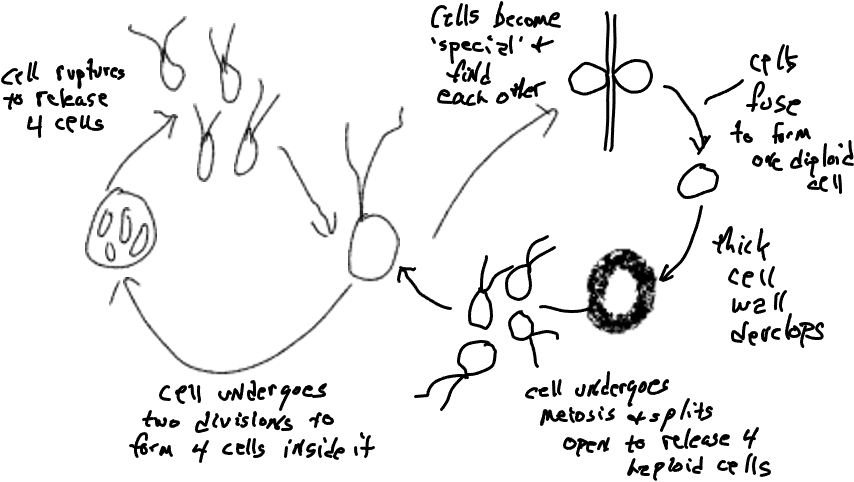
Oedogonium
Oedogonium, a filamentous green algae, shows the same basic patter as Chlamydomonas except that: (1) it is colonial, not unicellular, (2) in the sexual cycle the gametes do not look alike but are structurally very different with one (called the egg) being large and immobile and the other (called sperm) being much smaller and mobile. Sperm are released from the colonial filament to swim to and join with the egg (syngamy). Both the egg cell and cell producing sperm need to develop a hole in the cell wall in order for the sperm to escape and enter the egg cell. The zygote develops into a zygospore that is released from the filament and eventually undergoes meiosis and releases flagellated zoospores that, like the asexual zoospores, are capable of attaching to a substrate and developing into a filament, i.e., the cell that initiates a colonial organism is not a diploid zygote, rather it is haploid zoospore.
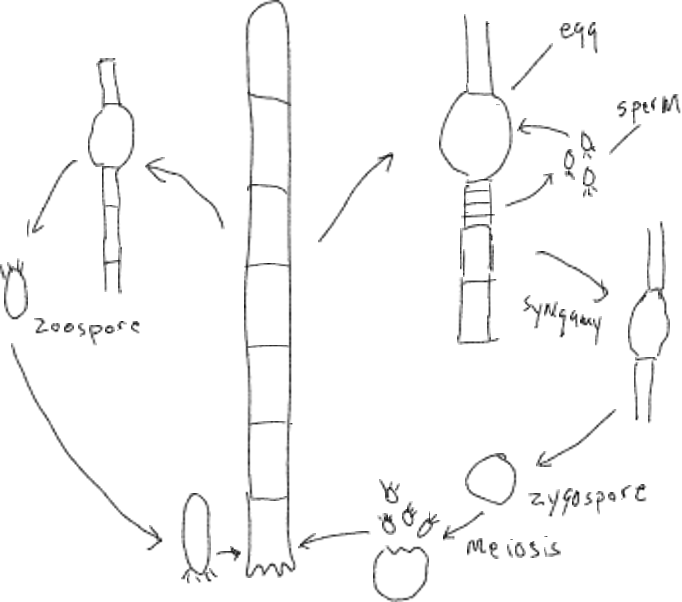
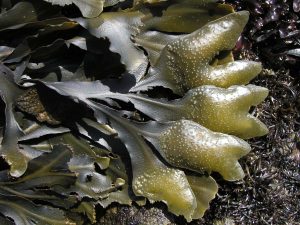
Fucus
Fucus is a multicellular brown algae with a life cycle comparable to humans (Fig. 7-9). The organism is diploid and the only haploid cells are gametes, which come in two varieties: a large, unflagellated egg and a small, flagellated sperm. Unlike in humans, gametes are released to the environment and syngamy occurs there. The zygote is formed outside of a parental gamete-forming organism. The zygote develops into a new diploid organism, which, unlike humans, generally can produce both male and female gametes. Note that, in contrast to the two previous examples, the zygote divides mitotically and does not undergo meiosis, hence there is a diploid organism, not simply a single diploid cell.
- Fig. 8 Cross section of a conceptacle, showing the opening to the outside (to the left of A) and the structures that produce eggs (C) and sperm (D).
- Fig. 9 Diagrams of the egg and sperm producing structures. Eggs are produced when a diploid cell undergoes meiosis with the four haploid products dividing mitotically to produce eight eggs that are released; sperm are produced when a cell undergoes meiosis followed by four rounds of mitosis to produce 64 flagellated sperm that are also released.
Saprolegnia
Saprolegnia, a water mold in the same group as Phytophthora, also has a life cycle that is primarily diploid, like humans. The organism is filamentous and siphonaceous, with no cross walls. The nuclei found in the hyphae are diploid and the the diploid organism is capable of reproducing asexually by producing structures that produce diploid, flagellated zoospores that are mobile and capable of germinating to produce more diploid organisms. This is the primary way that the organism reproduces. The zoospores may also develop into cysts, dormant cells, capable of surviving conditions that the zoospores and hyphae cannot. The diploid hyphae may also be induced to form enlarged spherical structures at their tips that contain a single diploid nucleus that undergoes meiosis. After a few additional divisions the structure now contains several haploid eggs. Chemically attracted to the egg containing structures are hyphae that develop enlarged hyphal ends that attach to the egg producing structure and whose diploid nuclei to undergo meiosis to produce sperm. The structure also produces fertilization tubes that penetrate the egg containing structure and allow the haploid nuclei (‘sperm’) to enter and fertilize eggs. These zygotes develop substantial cell walls and are termed oospores. These remain dormant and are capable of dispersal and survival under adverse conditions. When they germinate they produce diploid hyphae.
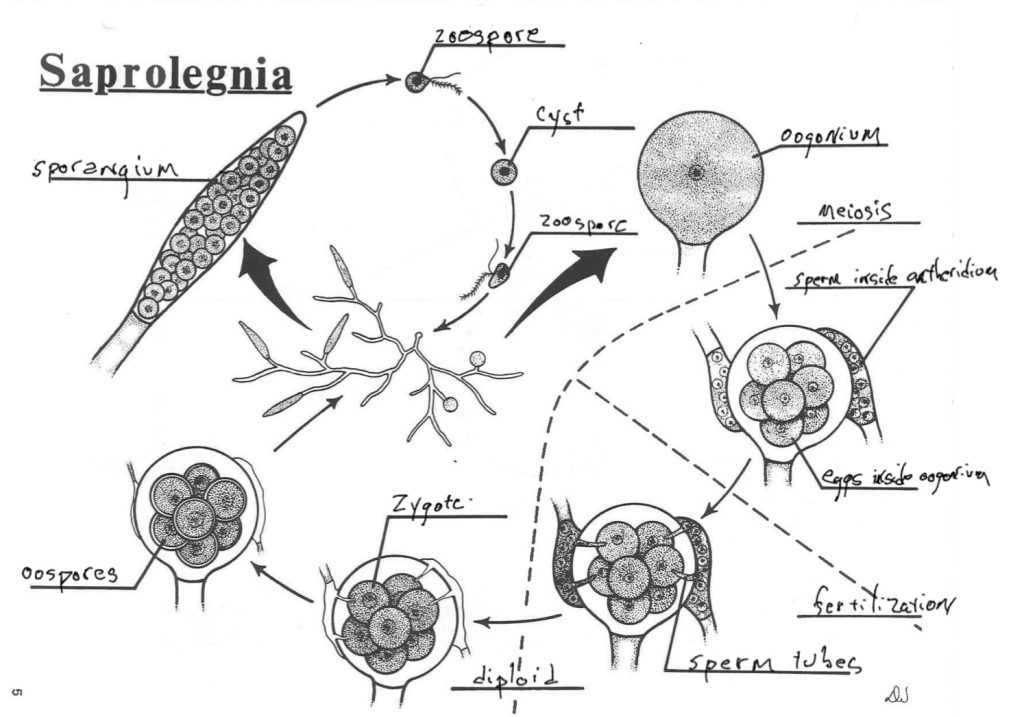
Laminaria
Laminaria is an extremely large multicellular brown algae with a life cycle that departs slightly from that of humans and Fucus in that the products of meiosis (called meiospores) are not gametes and are not capable of fusing with each other. Instead they are a type of ‘spore’, a cell that, like some zygotes, is capable of developing, in this case developing into a microscopic filamentous colonies that do produce gametes. This is similar to what happens in Oedogonium. In Laminaria there are two types of meiospores that develop into two different types of filamentous gamete-producing colonies. These are not distinguishable by form but one produces eggs and the other produces sperm. The sperm are released to the environment and are attracted to and fertilize the eggs, which may or may not have been released (if it has not been released then the filament that produces it deteriorates soon after fertilization). The zygote attaches to a substrate and grows into the large kelp that is typical of the species.
Laminaria exhibits what is called alternation of generations, (Fig. 11) producing two different organisms, one diploid, one haploid. The haploid form produces gametes and is called a gametophyte. The diploid organism produces spores and is called a sporophyte. Note that both spores and zygotes show ‘developmental potential’. Spores divide and produce a haploid colonial filament (the gametophyte). Zygotes divide and produce a diploid multicellular kelp that is initiated in the female gametophyte that has produced the egg. Since the haploid and diploid forms are very different, Laminaria’s life cycle is described as ‘heteromorphic alternation of generations’ with hetero- referring to different and -morphic referring to forms.
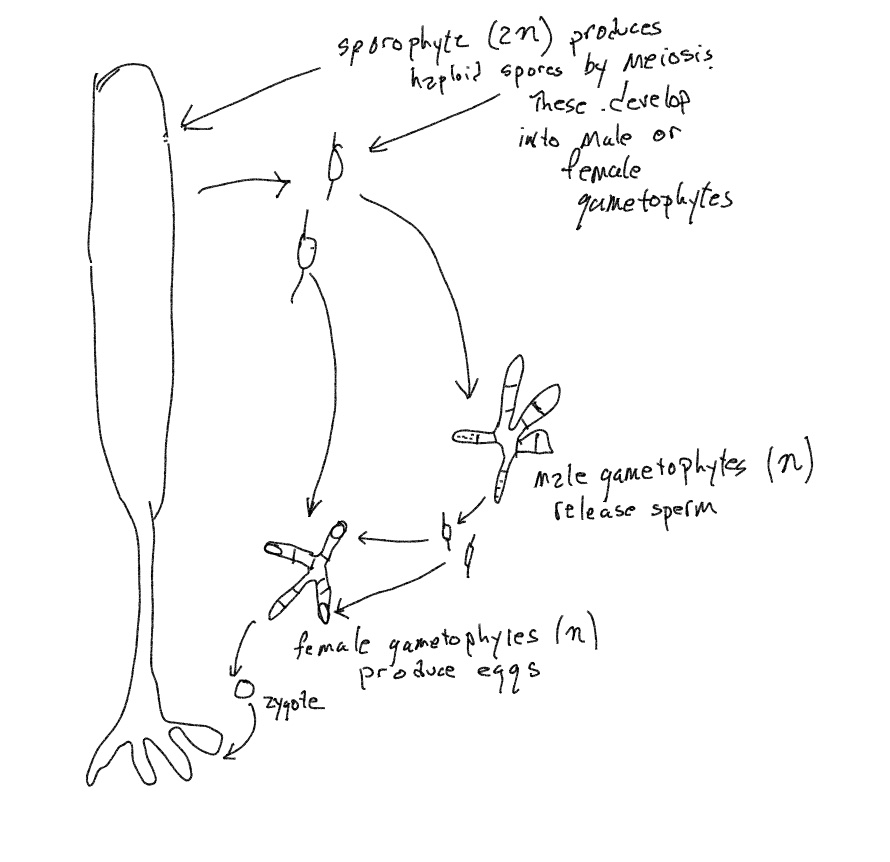
Ulva
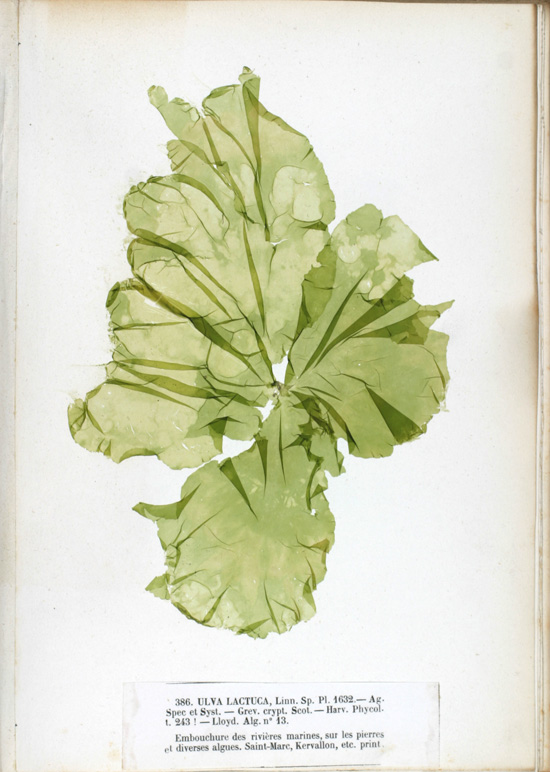
Ulva is a colonial green algae, producing sheets that are two cells thick. It produces two forms, one haploid, one diploid, that are indistinguishable in form. Diploid organisms are produced from the growth of zygotes produced by the union of two gametes. The gametophytes (i.e. the haploid organisms that produce gametes are produced by the development and growth of haploid spores; these are products of meiosis that occurred in cells at the margins of the diploid plants, which are sporophytes. Diploid organisms are produced by the development of zygotes (note that, like Laminaria, both haploid spores and diploid zygotes show ‘developmental potential’; they can develop into organisms). Ulva demonstrates what is known as ‘isomorphic alternation of generations’ (iso- referring to same); the gametophyte and sporophyte look the same.
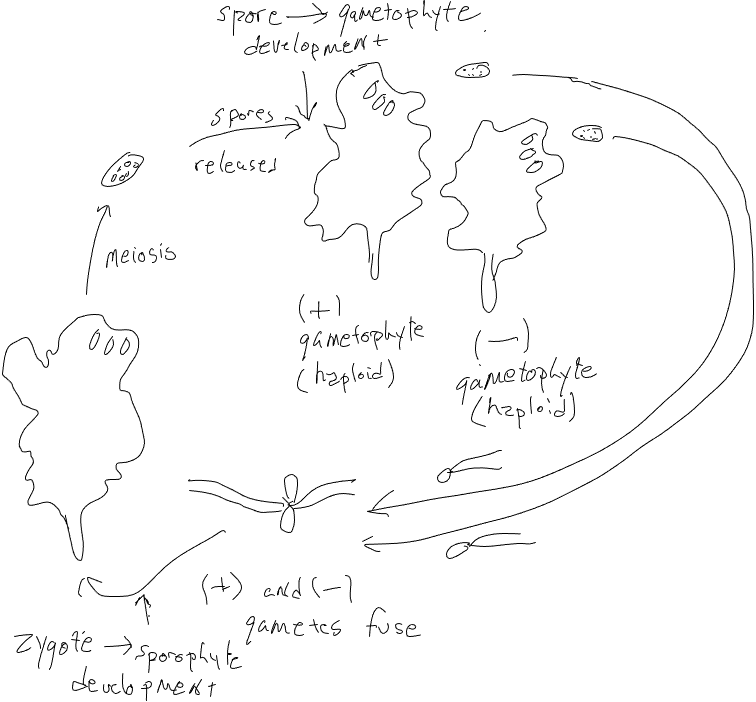
Porphyra
Porphyra is a red algae that, like Laminaria, has two forms that look very different. The haploid, gamete-producing form (i.e., the gametophyte) (Fig. 14) is a macroalgae and has long been harvested as food in both Ireland and Japan (nori). It is a sheet that may be one or two cells thick. The gametophyte form releases sperm that are unusual because they lack flagella (there are no flagellated cells in the red algae). The eggs are fertilized while still present on the gametophyte. The zygotes (called carpospores) are then released to the water column, settle and establish themselves by drilling into oyster shells or other substrates. They then grow into small, filamentous diploid, spore-producing organisms (sporophytes). The spores that are produced are diploid but undergo meiosis upon germination and grow into the larger, blade-forming gametophytes. In general, this life cycle is like that Laminaria, there is a heteromorphic alternation of generations, but the larger, conspicuous form is haploid and the smaller, inconspicuous form is diploid. One interesting aspect of the Porphyra life cycle is that both the gametophyte and sporophyte are capable of reproducing asexually. Another interesting aspect is that until the studies of Kathleen Drew Baker, published in 1949, the diploid organism was described as a separate red algal species. Her work was fundamental to the development of commercial Porphyra cultivation.
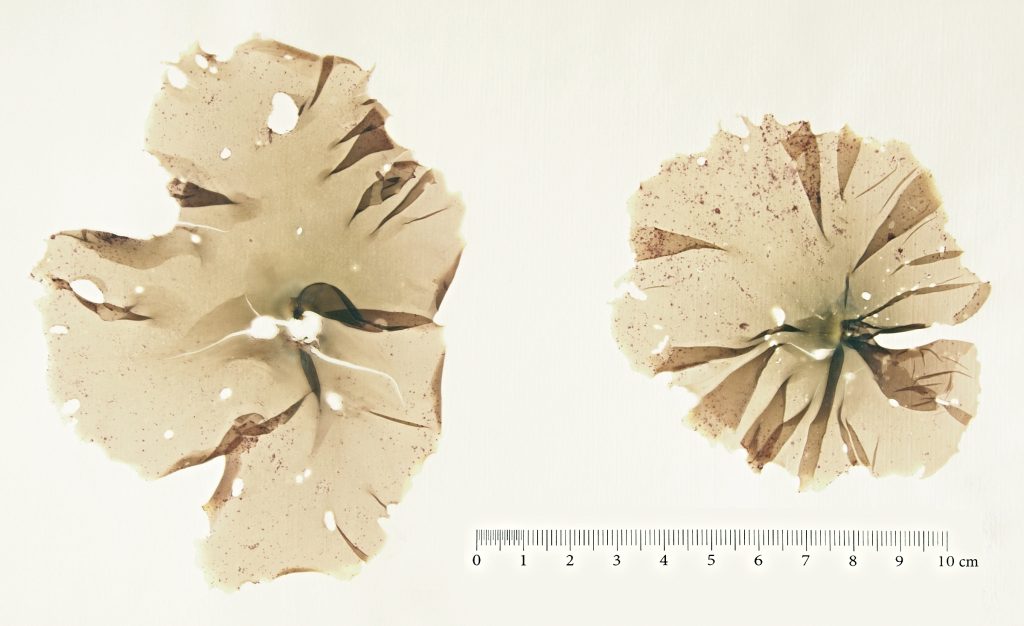
Polysiphonia
Polysiphonia is a red macroalgae with a finely branched form that looks identical as: 1) haploid, sperm-producing (male) gametophyte plants, 2) haploid egg-producing (female) gametophyte plants and 3) diploid spore-producing plants (sporophytes = tetrasporophytes). As in all the red algae, sperm do not swim but are carried by water currents after being released from the male gametophyte plant. If they are able to attach to a projection extending from the eggs, which are produced on the female gametophyte plants, then fertilization (syngamy) occurs. The zygote then develops on the female gametophyte plant, producing a very diminutive ‘extra’ diploid generation called a ‘carposporophyte’, that is found on the gametophyte plant. These produce diploid carpospores that are released and germinate to form what is called a tetrasporophyte plant. These look identical to the gametophytes but are diploid. The tetrasporophyte produces haploid spores as a result of meiosis and these germinate and grow into haploid gametophytes.
The basic pattern is an isomorphic alternation of generations but with an additional generation between the gametophyte and sporophyte (Fig. 14). Hence there are three ‘generations’, not two. Two of them are diploid and one is haploid. Several commonalities with other groups can also be pointed out, keeping in mind that none of these reflect phylogenetic relationships:
- the zygote is retained on the on the organism that produces the egg; an analogous situation is found in the life cycle of all plants and some green algae
- one generation, the carposporophyte, is very diminutive and completes its entire life as part of the organism that produced it; something comparable occurs in seed plants and some non-seed plants
- the egg produces a filamentous extension that facilitates receiving sperm; a similar structure is found in some of the basidiomycete (club) fungi, specifically the rusts, and, although they don’t have ‘typical’ sperm, in the ascomycete fungi. Similarly, although it does not directly involve egg and sperm, but rather the structures that contain the egg and sperm, a comparable structure is found in the stigma of flowering plants.
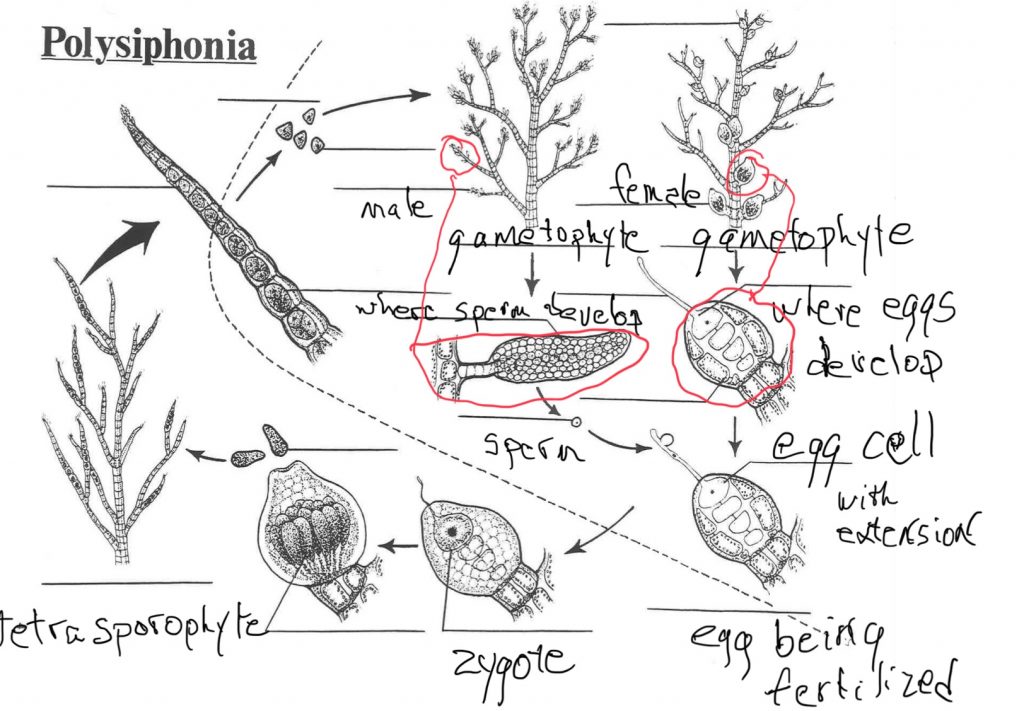
Further Reading and Viewing
- “Australian Bryophytes – Splash cups” by Heino Lepp. More on splash cups.
- “Australian Bryophytes – Elaters in liverworts” by Heino Lepp. Elators in bryophytes.
- “Splash-cup plants accelerate raindrops to disperse seeds” by Guillermo J. Amador et al. Splash-cup plants accelerate raindrops.
- “Size matters for violent discharge height and settling speed of Sphagnum spores” by Sebastian Sundberg. Spore discharge in sphagnum.
- “The Seaweed Site: information on marine algae – Fucus” by M.D. Guiry. Good site on “seaweeds” including rockweed sex.
- “Living together and living apart: the sexual lives of bryophytes” by David Haig. Bryophyte sex.
Media Attributions
- Budding in brewer’s yeast © Rosser1954 is licensed under a CC BY-SA (Attribution ShareAlike) license
- Plantlets at the margin of the leaf © Dr. Ahmed Elkordy
- Bulb bearing fern © profhorti
- Marchantia polymorpha thallus and gemmae cups © Rosser1954 is licensed under a CC BY-SA (Attribution ShareAlike) license
- Fucus © Steve Lonhart is licensed under a Public Domain license
- saprolegnia labelled
- laminaria new
- Ulva Lactua © Pierre-Louis Crouan is licensed under a Public Domain license
- Porphyra umbilicalis © Luis Fernández García is licensed under a CC BY-SA (Attribution ShareAlike) license
- polysiphonia labelled