Chapter 27: Biotic Interactions
Interactions between individual organisms
Organisms interact with each other and these interactions can have significant consequences to the participants. Most students are familiar with the classification scheme below which organizes interactions into types based on the consequences of the interaction on the two participants:
|
effect on the ‘larger’ organism |
|||
|
(-) negative | (0) neutral | (+) positive | |
effect on the ‘smaller’ organism |
(-) negative | competition | amensalism | predation, herbivory |
(0) neutral | amensalism | ‘neutralism’ | commensalism | |
(+) positive | parasitism | commensalism | mutualism |
There are multiple problems with this scheme and the definitions that stem from it. Larger vs. smaller is sometimes an arbitrary distinction. It is not clear what level, organism or population, it is focused on or how effects might be measured. At the level of individuals ‘ positive’ might be monitored by organism size, growth rate, longevity or reproductive success. But at the level of populations one might monitor population density or population growth rate. Sometimes what is ‘positive’ and what is ‘negative’ may not be obvious. A bird eating a poisonous butterfly is negative to both the individual butterfly and the bird, but at the population level one could argue that it is good for both the bird and butterfly populations. Some fungi living in plants (endophytes) substantially increase the growth of plants that they infect (positive effect), yet at the same time reduce or eliminate the likelihood of producing offspring (negative effect). Nitrogen-fixing bacteria infecting roots may benefit plants (faster growth, bigger plants) if soil nitrogen is low, but harm plants (slower growth, smaller plants) if nitrogen levels are high. Pollinator visits may benefit plants if they transport pollen to other members of the same species but not if their next visits are to different flower species.
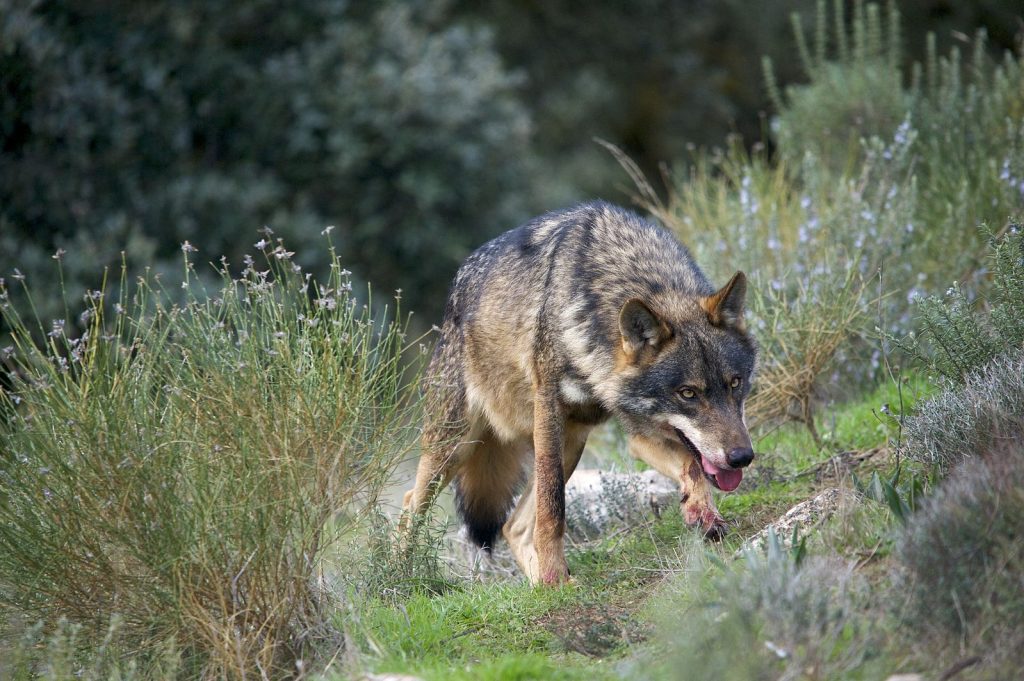
Perhaps the most significant biological context for the terms would be evolutionary (positive = enhanced reproductive success) but this may depend on circumstances that are difficult to evaluate. Predators (Fig. 1) would generally be thought to have a negative on prey populations, but in a number of situations, predators are thought to ‘benefit’ prey populations by preventing overpopulation. Seed predation (e.g., Clark’s nutcracker eating pine seeds, Fig. 2)) is clearly harmful to the individual pine organisms (i.e., the embryonic pine individual present in a seed) but apparently benefits the pine populations by allowing for dispersal. In short, the terms defined in Table 1 are not always useful.
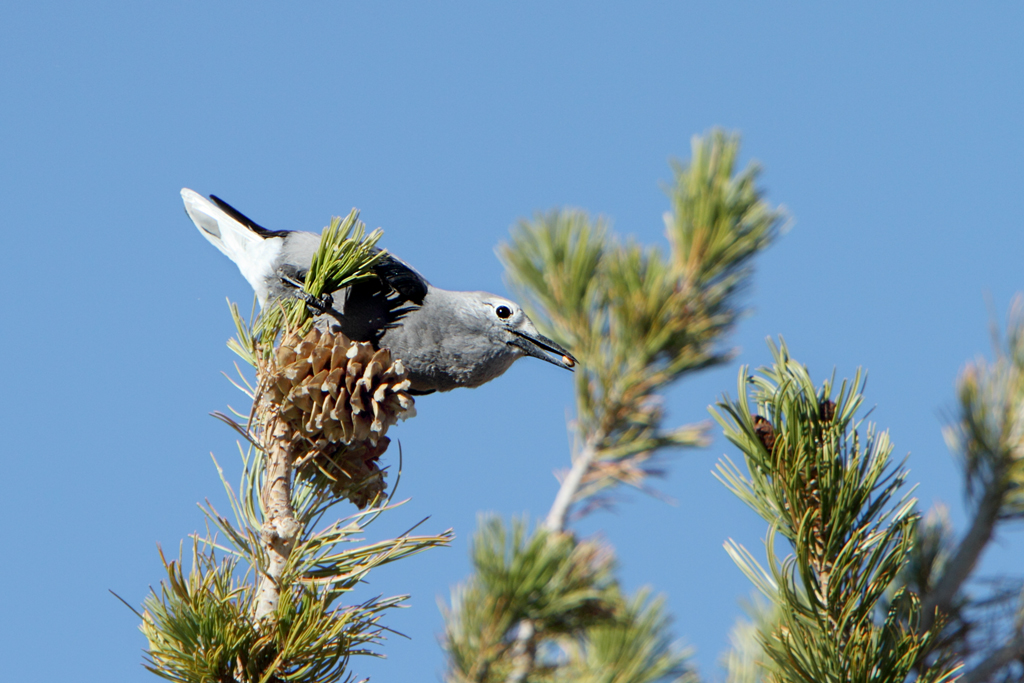
Another way to organize biotic interactions is not based upon arbitrary considerations of what ‘benefits’ or ‘harms’ the organisms/populations involved, instead, it is based upon the medium through which the interaction occurs:
- trophic interactions—one organism eats another or part of another, obtaining material (carbohydrates, proteins, fats) that can be used both for energy (i.e., burned in cellular respiration) or partially broken down and reformed into molecules of the consumer
- resources/conditions interactions—Resources are materials (e.g., oxygen) that an organism either produces, making them available for other organisms, or depletes/consumes, making them less available for other organisms. Conditions are physical parameters, e.g., pH, temperature, humidity, light intensity. Conditions influence organism behavior, and organisms can change conditions and thereby affect other organisms. Resources and conditions are combined here because several can be considered both as a resource and a condition. Oxygen can be considered a resource because oxygen can be produced or consumed but it also is a condition that has physical consequences, e.g., oxygen concentrations affect the solubility of ions in the soil solution. Similarly, light is a resource that plants ‘consume’, reducing its availability to the shaded plants below, but it also is a physical condition that influences all organisms in a number of ways.
- work interactions—one organism does work for another organism (work in the sense of physics and chemistry, a process that requires the expenditure of energy, e.g., moving material from one place to another, or producing a particular chemical or a physical structure).
TOPICS
- Trophic interactions
- Predation
- Grazing
- Parasitoids
- Parasites
- Leftovers
- Interactions involving resources and conditions
- Work interactions
Trophic Interactions
Although all trophic interactions are ‘organism A “eats” organism B’, resulting in a transfer of material from A to B, the way the eating is done varies greatly—most familiar organisms (i.e. animals) ingest into an internal tube where digestion occurs and the products of digestion are absorbed across membranes and into the eater. Most fungi digest outside and then also absorb digestion products. For both familiar animals and some fungi the process of killing of prey releases nutrients that can be absorbed with relatively little digestion. And a few organisms, e.g. blood parasites, tapeworms and some fungi, manage to get to and live in a place where nutrients are available without digestion.
As will be seen in some of the examples below it may be the case that the most significant consequences of the trophic interaction do not involve material gain and loss.
Below are four categories of trophic interactions. The categories are based on the manner of the interaction between the two ‘players’: the organisms doing the eating (‘eater’) and the organism being eaten (the ‘eatee’). In two categories, predation and parasitoids, the eatee is killed because of the interaction, while in the another two categories, grazing and parasitism, the eatee is not necessarily killed. And in a final category, (‘saprophagy’) the eatee was killed prior to the eating event. An assumption that holds most but not all of the time is that the eater always benefits. However, sometimes the material ingested is toxic or contains pathogens. As for the eatee, in predation and parasitoids it is clear that the individual is harmed, but, as mentioned above, the consequences may be different at the population level.
Predation
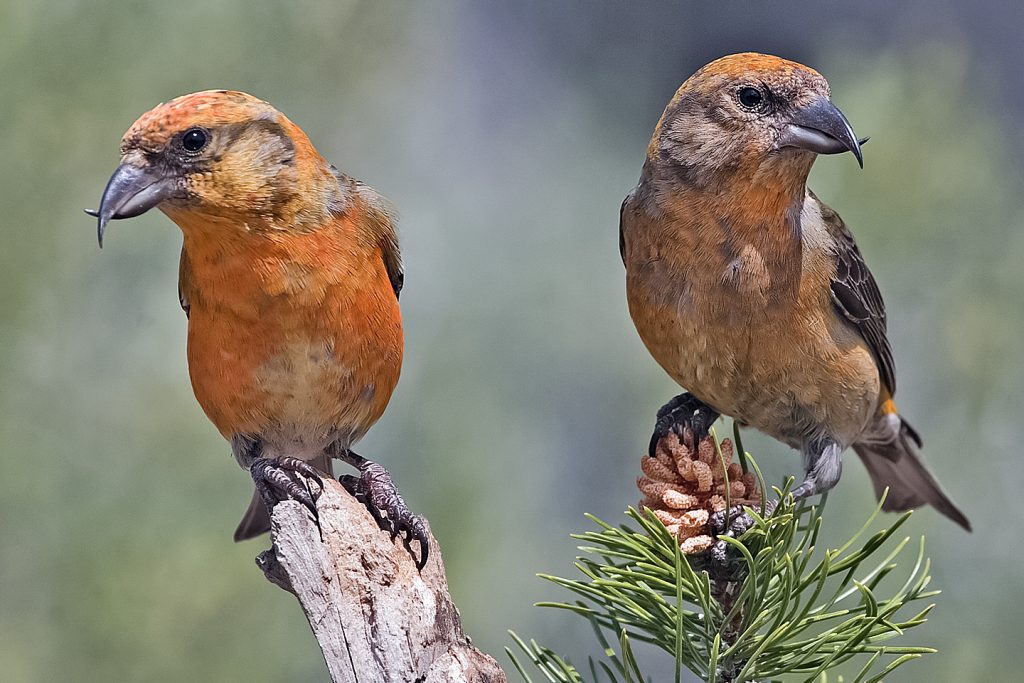
This trophic interaction kills prey (the ‘eatee’, the organism being eaten) and the eater, the predator, consumes multiple prey items during its life (Fig. 3). All forms of life (even predators) are subject to predation but for plants this most commonly occurs when the eatee is small: when they are pollen (tiny haploid male plants), seeds (‘seed predation’), or in the consumption of seedlings. The materials found in a pollen or seeds are particularly nutritious per unit weight because, compared to larger plants, they have relatively less structural material (i.e., cell walls) and relatively high concentrations of minerals and vitamins. Similarly, fungal spores as prey items are more nutrient rich than the hyphal strands. Predation is very important in aquatic systems where the base of the food chain are typically phytoplankton (unicellular photosynthetic autotrophs: cyanobacteria, diatoms, cryptophytes and unicellular green algae) that are preyed upon by zooplankton.
In predation the eater benefits from the nutrients and energy obtained (assuming that eatee does not contain toxins that the eater cannot handle) and the eatee is eliminated. For as long as seed plants have existed this interaction has driven evolutionary changes involving chemical, physical and phenological (timing) changes in plants, and consequent changes in predators. Particularly significant to plants is the fact that this interaction has developed into a means of dispersal, e.g. the consumption of pollen led to the development of pollination, with the eater transferring pollen from one plant to another. Although not as ubiquitous, a similar situation has developed into a mechanism of seed dispersal, utilizing an eater’s mobility and perhaps its caching behavior (see the reading on pines and the Clark’s Nutcracker). Both of these interactions are discussed below as ‘work’ interactions. Similarly, seed predation also was a driving force in the development of fleshy fruits as a means of seed dispersal: plants developed features that would reward the eatee in a way that did not involve (permanently) consuming the seed and killing its embryo. Seed predation also accounts for some fruit structures that protect the seeds inside (e.g., nuts), although seed predators have responded by developing structures (sharp teeth of saki monkeys or beaks of goldfinch, Fig. 4) and behaviors that allow them to open seeds, or digestive systems that grind the seed coat or fruit coat away.
Although one hears of ‘carnivorous’ or ‘predatory’ plants, the names are misleading. These plants certainly do kill ‘prey’ but they do not obtain food from them, in the sense of materials that can be utilized in cellular respiration. Predatory plants are photosynthetic autotrophs and their food (carbon and energy) requirements are satisfied by photosynthesis. For these plants ‘predation’ is connected to mineral nutrition. This is discussed further below as involving changes in conditions.
There are some photosynthetic organisms that are also heterotrophic (i.e. they are mixotrophs). Both the euglena and dinoflagellate groups include predatory members, some of which are also capable of photosynthesis. [Note that the adjective ‘mixotrophic’ can be applied both to these two groups and also to specific organisms within these two groups, i.e. both these groups have three types of organisms: solely photosynthetic, solely heterotrophic, and members that can be both depending upon circumstances.] The predatory dinoflagellates and euglenoids capture prey via phagocytosis. In addition, some dinoflagellates kill prey with toxins and then digest and absorb them. And a few of each group could be considered both parasites and parasitoids, defined below.
Considering the solely heterotrophic groups considered in this text, cellular and plasmodial slime molds are sometimes predatory and a few fungi are predators in the classic sense (i.e., they capture prey and kill them, http://www.mykoweb.com/articles/FungalSnares.html). Although they don’t capture prey in the classic sense, plant pathogens that are described as ‘nectrotrophic’ (see Chapter 30) are predators that kill plants and then consume them. Examples include: Pythium, a water mold (Oomycete) that causes ‘damping off’ of seedlings; the ascomycete fungus that causes dutch elm disease; and the ascomycete fungi that cause wilt diseases in tomatoes. The pathogen may produce toxins that kill cells, and eventually entire organisms, or, in the case of the wilt diseases and of dutch elm disease, produce chemicals that plug the xylem tissue and eventually kill the plant by preventing water transport. Other pathogens of plants might be considered parasitoids or parasites, discussed below. But the majority of inanimate heterotrophs are saprophytes, also described below.
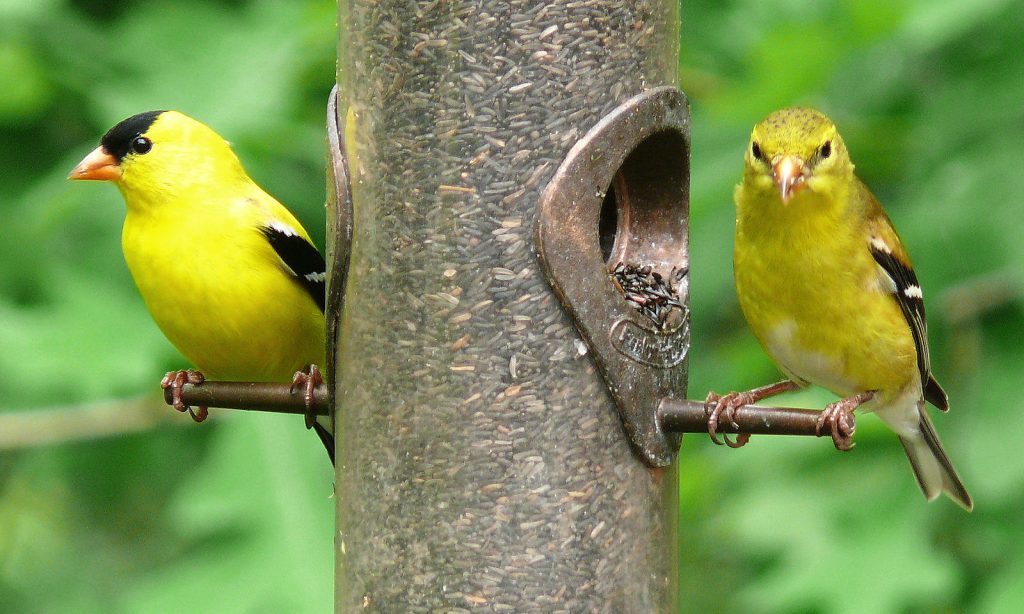
Grazing
The eater (grazer) does not kill the eatee, but only eats part of it, and usually, the grazer eats parts of several individuals. Most familiar examples of herbivory (eating of plants) are grazing: cows eating grass, deer eating shrubs, Japanese beetles (Fig. 5) eating ornamental plants. While most familiar grazing is on plants and on their leaves, organisms graze on other plant parts: stems, flowers, roots. And a lthough plants are the most common kind of organism
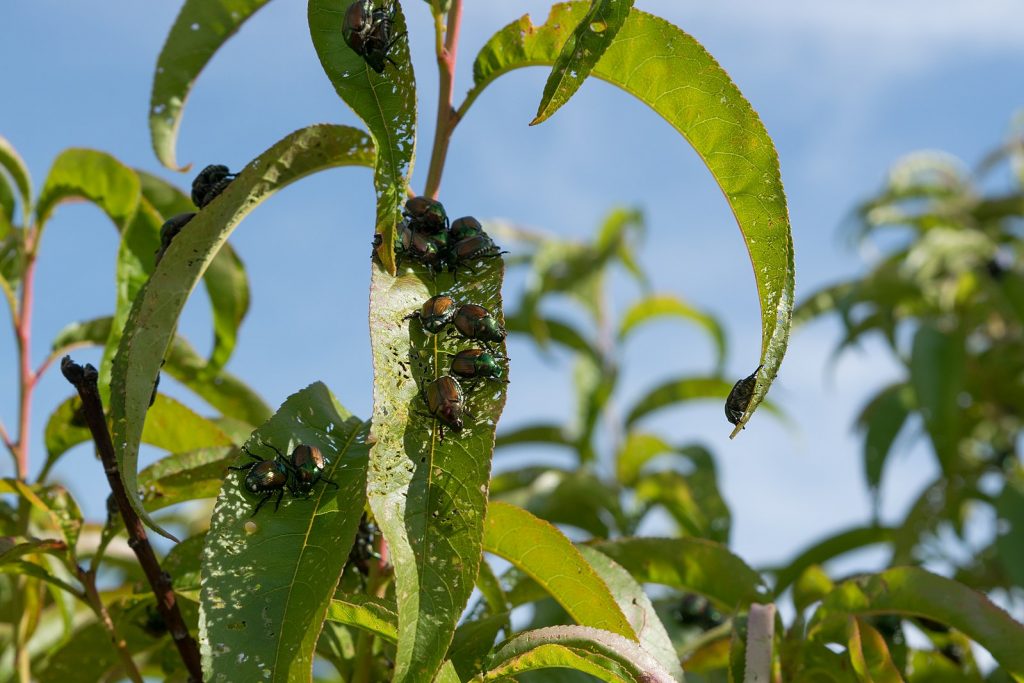
grazed upon there are several other ‘inanimate’ groups that are grazed: photosynthetic protists (‘algae’, in particular ones that are ‘large’ by being multicellular, colonial, or siphonaceous). A few non-photosynthetic protists e.g., plasmodial slime molds, and many fungi are also grazed. Grazing is most likely to occur on organisms that have indeterminate growth.
Grazing affects the eatee in two ways: by the loss of structure that is used to acquire matter and energy (e.g. leaf loss in photosynthetic plants), and also because of the loss of nutrients (e.g., nitrogen and phosphorus) that are removed by grazers and are relatively hard to replace. Too much grazing can be fatal for the eatee but lesser amounts of grazing sometimes actually produce ‘beneficial’ (depending upon definitions and perspectives!) results; for instance, grazing of apical meristems can induce branching and produce a plant that actually produces more leaves, flowers and fruits. Artificial grazing, i.e. pruning, is a very common horticultural technique to improve yield and quality.
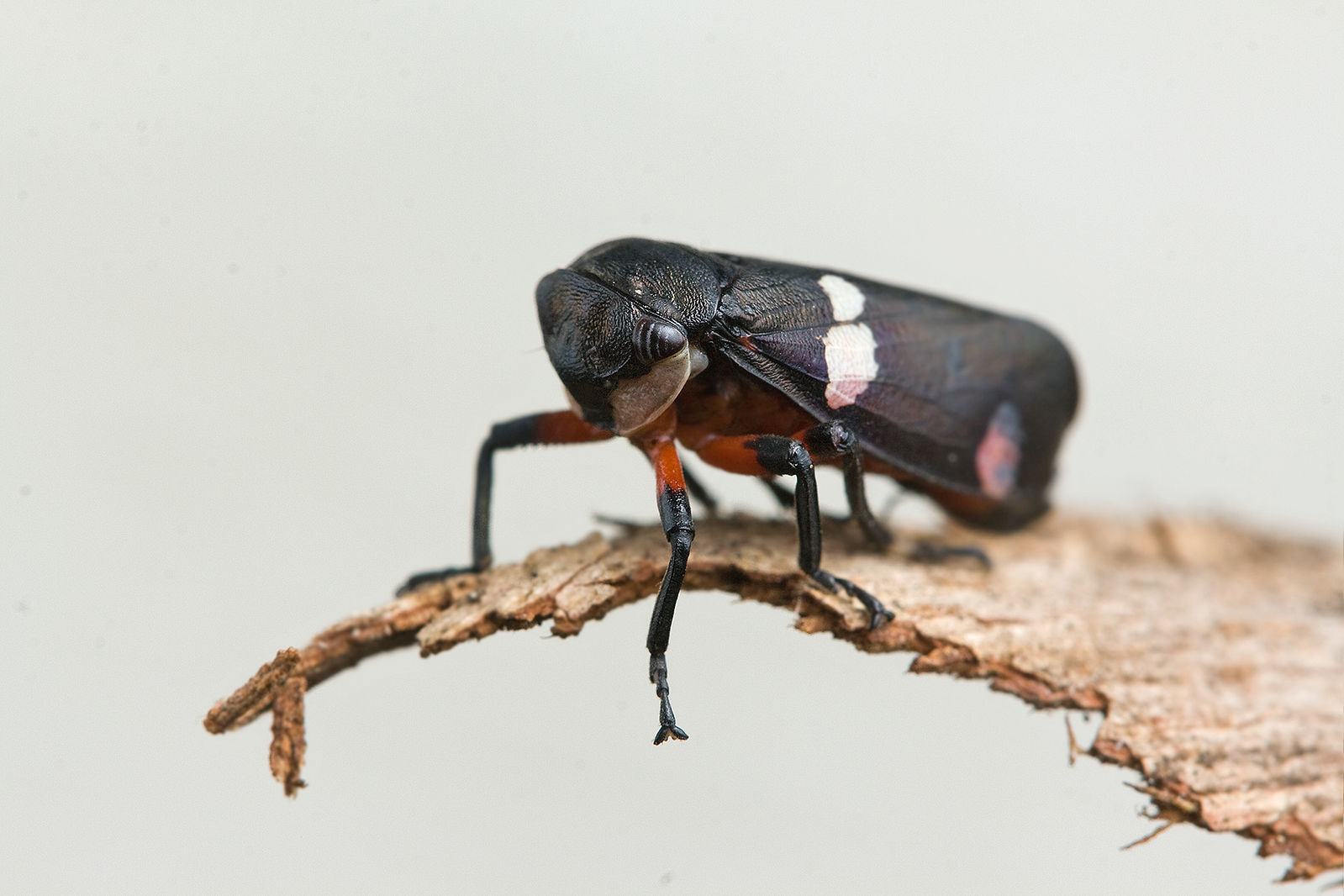
One particularly significant aspect of grazing is that it is a means of disease transmission between individuals, such as a mosquito (a grazer) spreading malaria. Leafhoppers and aphids are both insects that have piercing mouthparts to acquire nutrients from phloem tissue in leaves and stems. If they move from an infected plant to another plant they can transmit pathogens (viruses, bacteria and others) in the process, and often this is the most significant aspect of their grazing (Fig. 6).
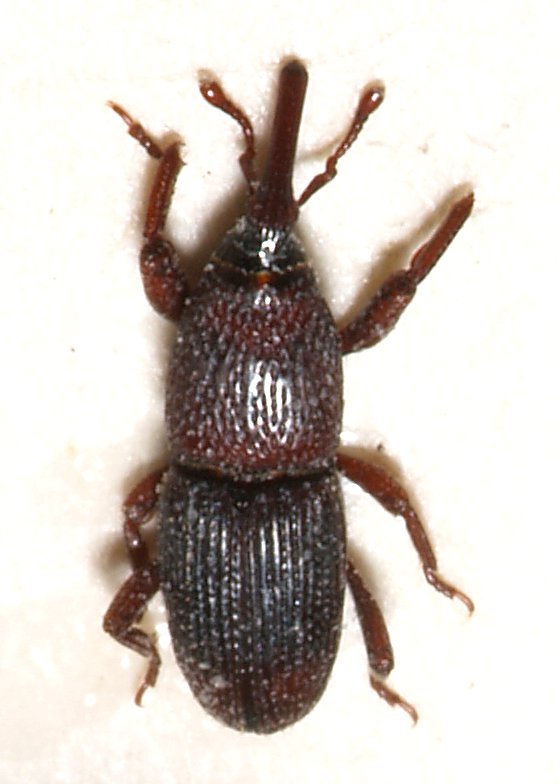
Parasitoids
The distinctive feature here is that the reproduction of the eater is obligately and directly associated with the trophic event and also that the eatee dies as a result of the association. While any heteterotroph must consume food in order to acquire the matter and energy needed to reproduce, in the case of parasitoids the connection is direct because a propagule (usually a fertilized egg) is deposited on the eatee. Another feature of parasitoids is a determinate life cycle where the final stage of development involves reproduction and the demise of the host (cf. to parasites, discussed below). The vast majority of parasitoid interactions involve insects, one as the eater and another as the eatee. But there are a number of cases from the realm of inanimate life. Some dinoflagellates could be considered parasitoids on nematodes. And a number of fungi that are described as ‘entophagous’ (= insect eating) are parasitoids. Probably the most famous one of these is Entomophaga maimaiga, that is used as a biocontrol agent for gypsy moth. Spores of Entomophaga germinate on the caterpillars of gypsy moth, grow through the cuticle and produce a mycelium that consumes the entire caterpillar and eventually produces condidia that are dispersed through the air to other caterpillars. In addition to being predators on nematodes there are some fungi that could be considered parasitoids on nematodes, the distinction being that predators capture and kill prey and parasitoids ‘enter’ and then kill prey. There are also some chytrids that might be considered parasitoids, with some attacking both pollen and unicellular algae (phytoplankton). Interestingly, the zooplankton that normally feed on phytoplankton may switch to eating chytrids, thus there appears an additional link in the food chain (phytoplankton–>chytrids–>zooplankton). Another fungal group that could be described as parasitoids are some of the smut fungi. It should be noted, however, the range of lifestyles present in fungi makes the predator/parasite/parasitoid distinctions challenging.
A final parasitoid interaction to mention, because it is sometimes economically significant, involves insects eating seeds e.g., granary weevils (Fig. 7) and acorn weevils, where adult insects deposit their eggs in seeds (see this short video by National Geographic on the acorn weevil: https://vimeo.com/38056185).
Parasites
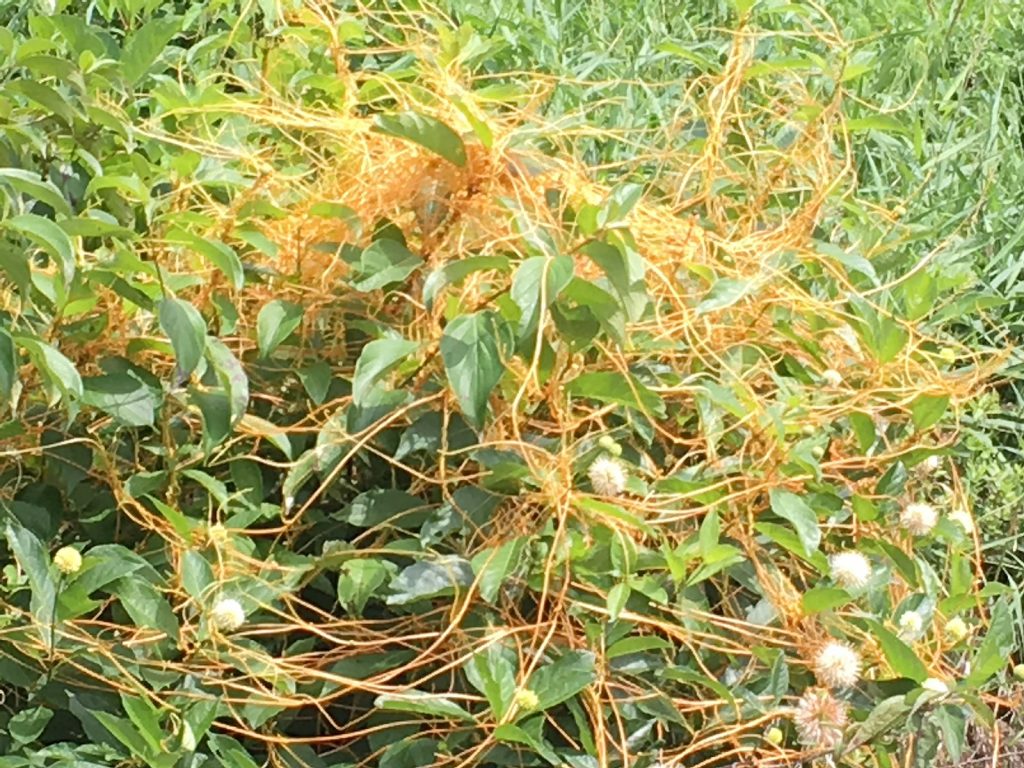
In parasitism, as in grazing, the eater generally does not kill the eatee, but in contrast to grazing, the eater generally only feeds upon one host and thus is not typically a means of spreading disease from one eatee to another. Evolutionarily, one might argue that parasites (and perhaps some grazers) adopt a strategy of keeping their host alive in order to assure a food supply for a longer time. Going one step further, it may be beneficial for the eater to make the eatee more vigorous, which is the case with mycorrhizae where fungi feed off their hosts but somehow allow them to grow more vigorously. Thus, although the eater in parasitic relationships always benefits because of the material gained, the effect on the individual eatee can range from increased longevity and vigor, through indifference (with no apparent influence on the longevity of the host), to definite adverse effects resulting from lost material and sometimes ultimately resulting in death. Looking at the classic scheme (Table 1) the bottom line depicts the multiple consequences of this type of trophic parasitism. The term parasite is also applied to situations that do not involve feeding (see the work interactions discussed below). Parasitic interactions abound and nearly all of the inanimate groups covered in this text are at times both the eatee and the eater. A few of the interesting cases are discussed below.
Red algae parasitize other red algae, often the parasitizing a close relative, a situation that is also common in insects. There are brown algae that parasitize other brown algae. There are green algal parasites of insects and dinoflagellate parasites of crabs. It is also interesting to note that the apicomplexa, a group that includes Plasmodium, the causal organism for malaria, along with other significant parasites of humans, are considered to be derived from a group of pigmented ancestors that also gave rise to the red algae and the dinoflagellates. Fungi are often parasites on a variety of hosts, notably plants and other fungi. And even some plants are parasites, both on other plants as well as on fungi, examples below.
There are a number of fungi called biotrophic fungi (see Chapter 30), including the important plant pathogens powdery mildews and rusts, that feed off of living plant tissue and would be considered parasites. They produce structures called haustoria that penetrate the cell wall and interact with the plant cell plasma membrane, providing them with access to materials (e.g., sugars, amino acids) present in the cytosol. Dodder (Fig. 9), a non-photosynthetic flowering plant, also produces haustoria. These penetrate into the phloem tissue of their hosts and provide them with nutrition. T he fungal component of mycorrhizae would be considered parasites, as would be the nitrogen-fixing bacteria that form galls in some plants. Indeed, most gall-forming organisms (insects, fungi, mites) would be considered parasites. The gall is an abnormal growth induced by the presence of the parasite. The parasite is fed by its host and ultimately exits the gall.
As an example see “The Amazing Tale of Sagebrush Galls” by Susan Ballinger at www.wenatcheenaturalist.com/sagebrush-galls/ or “Inside the Goldenrod Gal” on the Finger Lakes Land Trust blog at www.fllt.org/inside-the-goldenrod-gall/. Most of these interactions are similar to parasitoids in that the reproduction of the eater is dependent on the interaction; however, the host is not killed. In most instances, the eatee suffers because of a loss of nutrients, and the eater benefits because it is provided with nutrition and often with protection as a result of the structure of the gall.
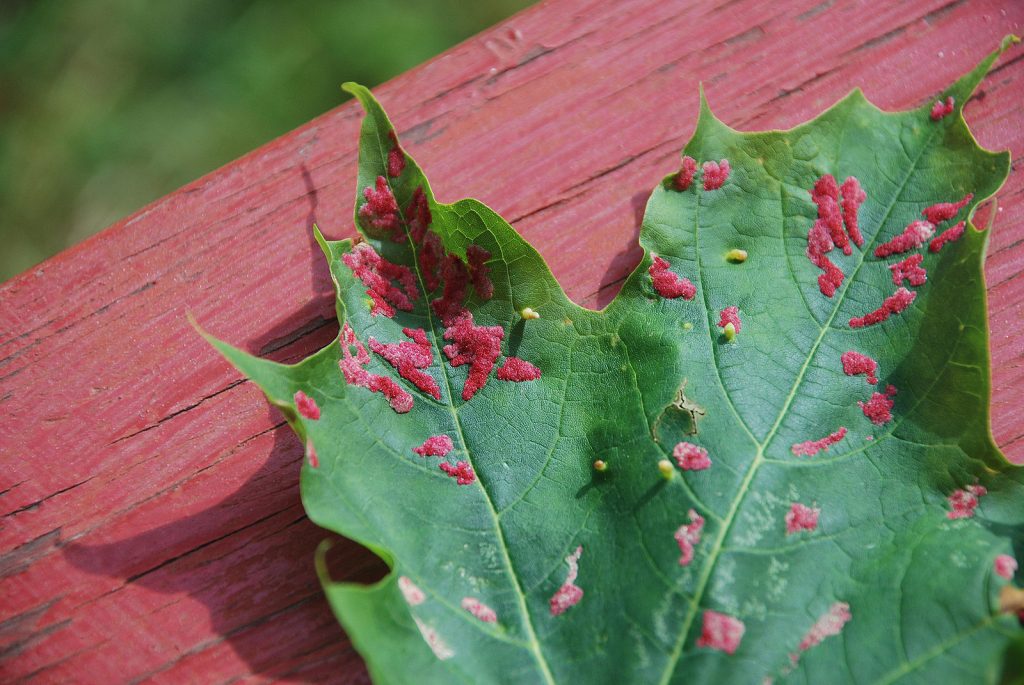
Saprophytes, i.e., eating ‘leftovers’
All organisms are a source of food after they die and thereby represent an ‘easily captured’ prey item. Heterotrophs that consume material (plant, animal, fungal and others) that is already dead (i.e., they did not kill it) are termed saprophytes. Because plants are continually shedding leaves and roots (and in many cases stems), these discarded items are often abundant and provide food for a number of organisms. The nutrient quality of these discarded items is generally substantially below that of living tissues because the plant, in the process of senescence, recycles materials, retrieving much of the material present in the soon to be discarded item before it is actually shed. Leaf protein, nucleic acid and mineral concentrations all decline drastically before leaves are shed and the remaining material is much less digestible both because most of the molecules that remain (e.g., cellulose, lignin) are more difficult to degrade and because of the lack of nitrogen makes it hard to build up substantial populations of saprophytes. Organisms (especially larger ones. e.g., earthworms, insect larvae) feeding on dead organic material often obtain nutrition, perhaps most of their nutrition, from the consumption of much smaller organisms (e.g., bacteria, amoebae, fungi) that are present on the decaying material and not from the organic material itself, i.e., they are really getting their nutrition from being predators, not saprophytes. Bacteria and fungi are the most significant saprophytes in the soil but other groups (cellular slime molds, plasmodial slime molds and heterotrophic forms of euglenoids) may also be saprophytic. In aquatic systems, a significant amount of organic material can be broken down to the point that it dissolves and is present as dissolved organic material, which some organisms are able to assimilate.
Conditions/Resources Interactions
All organisms change the resources and conditions around them. The extent to which this affects other organisms depends on: how big the organism is (big things have more of an effect than small ones), how plentiful they are (i.e., their population size) and precisely what changes they bring about. Any organism is a ‘producer,’ i.e., it grows and produces a resource (i.e., biomass) that some other organisms can eat. Therefore all organisms must provide resources. Plants (and more generally autotrophs) are particularly important in a resource sense because, being the base of food chains, their activity represents how many trophic resources are available to entire communities. Also, except for autotrophs (who are ‘self-feeders’) all other organisms, i.e,. heterotrophs, eat something and can influence other species by depleting that resource. But there are many other ways that organisms interact with each other besides affecting trophic resources. Here are some examples:
The trees of a forest substantially change the conditions below them. Temperatures are moderated (cooler in the daytime, warmer at night). Wind is moderated. Rainfall/snowfall patterns are changed. For deciduous forests the annual deposit of leaves covers the ground surface and acts like insulation, keeping the soil warmer in the fall and cooler in the spring. In evergreen forests, leaves layer the ground but the fact that they do not come all at once alters the dynamics. Leaf litter affects soil chemistry. Thus different species can have different effects. The tipping over of a tree root system that occurs when trees are blown over changes the topography and exposes lower mineral layers. And some species (e.g., yellow birch) germinate best in mineral soil because the roots of seedlings are unable to penetrate a blanket of leaf litter.
Oxygen consumption by organisms in terrestrial habitats generally has little impact because the oxygen levels in the air are high (~20% of the air is oxygen) and because winds keep the air well-mixed and localized depletions are unlikely. In contrast, oxygen depletion in the soil and in some aquatic situations can have very significant consequences. In waterlogged soil, oxygen consumption by roots, fungi and a host of soil organisms, coupled with reduced oxygen movement in because of the soil’s water-filled pores, can make the soil anaerobic. Similarly, unless there are processes promoting mixing of the water column, respiration by heterotrophs at the bottom of the lake, where organic matter settles, significantly lowers the oxygen levels, potentially affecting all organisms living there.
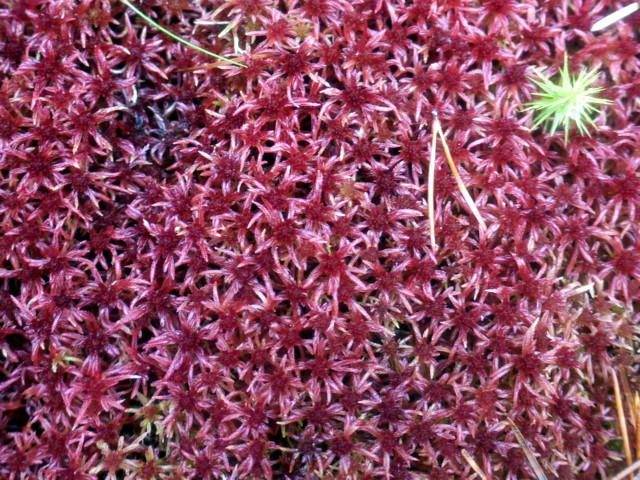
A globally significant example of organisms changing oxygen availability and consequently affecting other organisms involves Sphagnum moss and areas known as bogs. Sphagnum moss can develop high population densities and this, coupled with their pattern of growth, creates a dense mat of stems elongat ing at the top but with an extensive layer of dead stem and ‘leaf’ material below. The stems and leaves hold substantial quantities of water and water is also held in the spaces between plants, producing what is essentially a giant sponge. This creates a habitat of stagnant water, where there is little mixing of the surface layer of water with the water below. C onsequently, at a very shallow depth, the water becomes anaerobic because of oxygen consumption by saprophytes feeding on the dead plant material. In addition, the chemistry of sphagnum cell walls causes the water to become acidic. The acidic conditions, combined with lack of oxygen, greatly reduce the decomposition of plant material, thereby reduc ing the supply of nutrients (remember that nutrients become available to plants because of decomposition), and cause peat (un-decomposed plant material) to accumulate. The soil conditions prevent a large number of species (e.g., trees) from existing in the area or causes them to have stunted growth. Instead, bogs have a characteristic group of species, often members of the blueberry family, that are tolerant of the soil conditions.
One of the types of plants found in the nutrient-poor conditions of a bog are ‘carnivorous’ plants including pitcher plants, which nicely demonstrate the nutritional distinction between autotrophs and heterotrophs. Heterotrophs obtain both ‘food’ (a carbohydrate supply for cellular respiration) and nutrients (e.g., nitrogen) from the material they consume. Carnivorous plants obtain energy through photosynthesis just as most plants do, making food in photosynthesis and then eating it. The significance of their ‘predation’ is that it provides carnivorous plants with nutrients (not ‘food’) that are otherwise hard to come by because of the habitats where they dwell. There are a wide variety of devices for capturing prey (generally insects), including pitchers, snap traps, flypaper, bladders and lobster traps (see more carnivorous plants at https://earthsky.org/earth/lifeform-of-the-week-carnivorous-plants-are-out-for-blood).
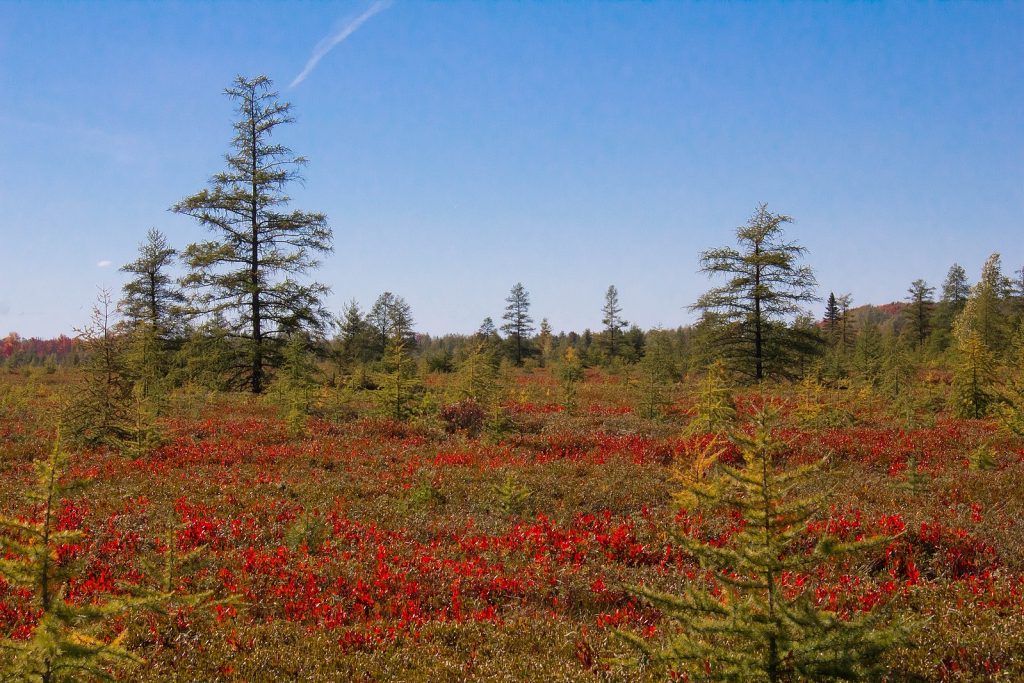
Pitcher plants have a highly modified leaf whose petiole serves as a container for rainwater. The pitcher also possesses features that attract and capture insects who eventually drown there. The water develops a decomposition community of bacteria, fungi, water molds, amoebae, insect larvae and others. As a result of their activity, nutrients become available in a forms that the plant can absorb. In contrast, nutrients do not become available to the roots due to the lack of decomposition in the waterlogged peat that pitcher plants are rooted in.
Dense growth of algal (usually cyanobacteria but sometimes green algae) can ‘seal’ the top of ponds, creating a situation comparable to sphagnum bogs because the wind is not able to induce mixing of the oxygen-rich top layers of the water with the lower layers. Because no light can penetrate the dense algal layer on top, no photosynthesis is possible except at the surface. These factors, coupled with the magnitude of dead plant material that is created by the algal growth and the decomposition of this material by heterotrophs below, cause the lower water levels to become anaerobic, eventually drastically altering the species present and limiting the rate of decomposition.
Resources /conditions represent significant avenues whereby species can interact with each other. In particular, two species requiring the same resource can affect each other by making the resource less available for other organism/species. Plants interact with other plants by their consumption of light, nutrients and water. This is the classic explanation for an interaction described as ‘competition’ although there are other reasons besides resource depletion that might explain why the presence of one species might deter the growth of another species (or at the population level, where the presence of one population lessens the population density of a second population).
Globally, two of the most important biotic consequences involving resources are the addition of oxygen to the atmosphere by photosynthetic organisms and the addition of simple mineral forms of nutrients into the soil or aquatic systems as a result of the decomposition carried out by heterotrophs.
Plants provide resources in other ways; their physical structure is important to many other other species: birds nest in trees; many spiders use plant structures as a base for their webs; parts of plants and pieces of lichens are often used as building materials for nests.
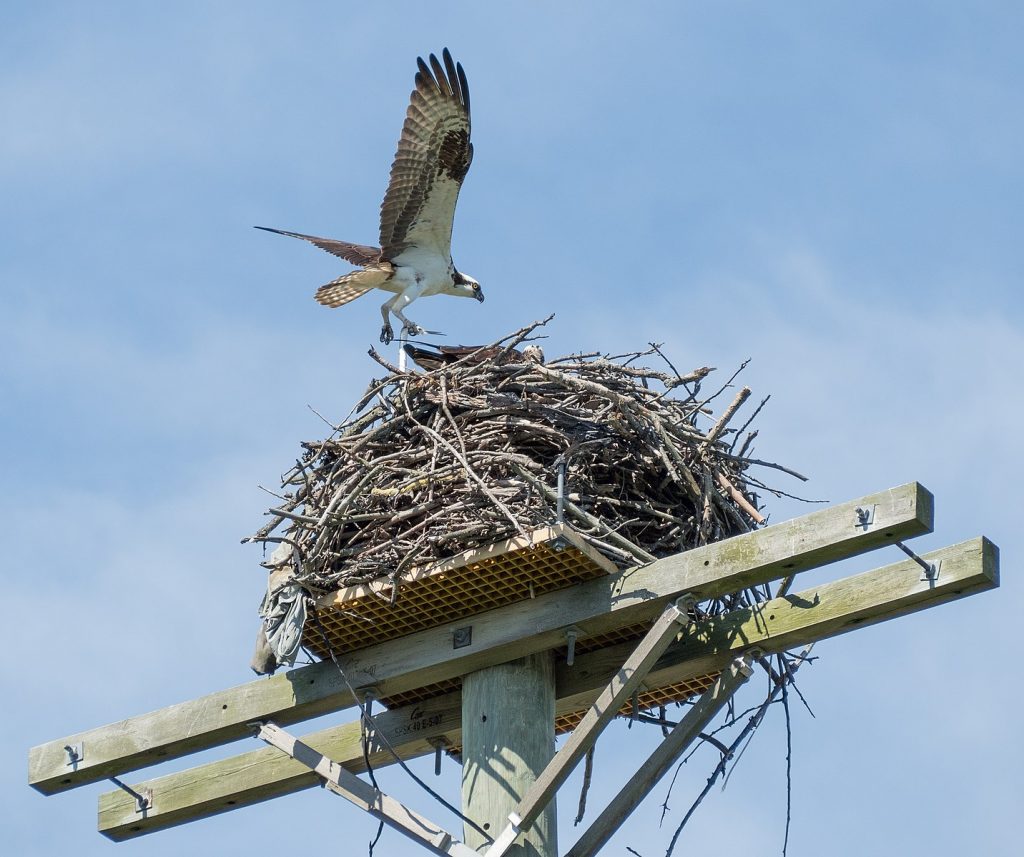
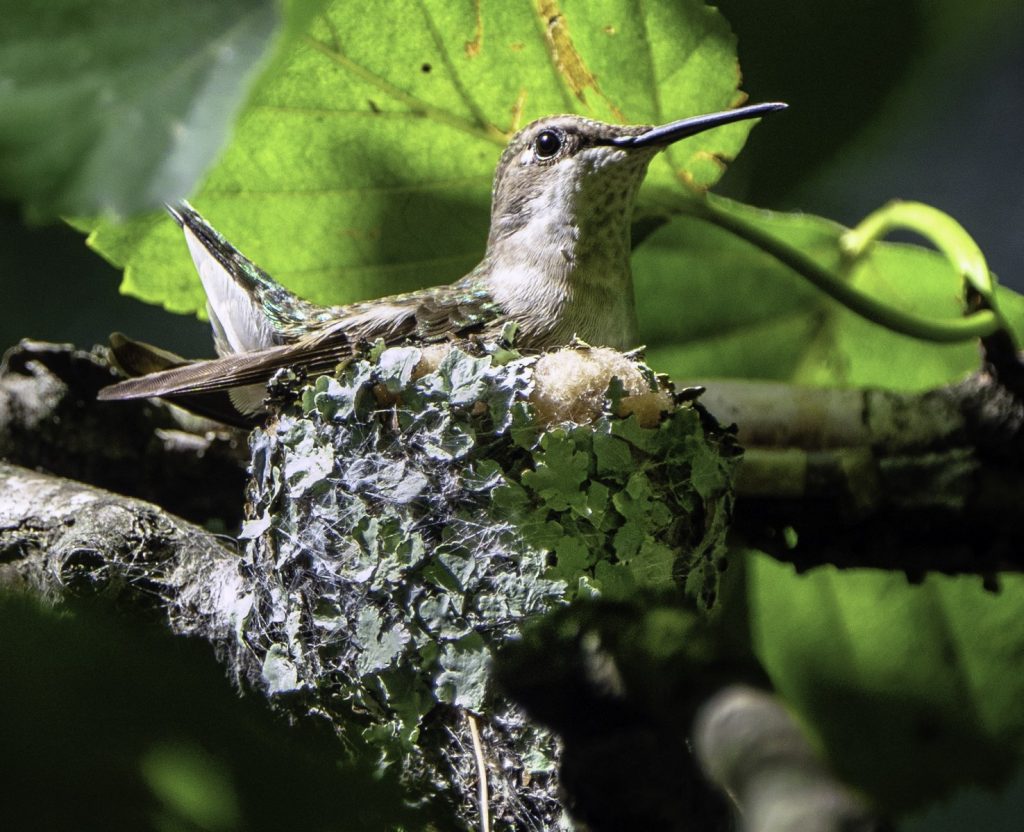
Work Interactions
A number of species interact with each other by providing services, i.e., doing work, for other species. For plants, the most significant of these results from organisms that provide mobility, mobility for male gametophytes in pollination and mobility for seeds. A nimals transport seeds both ‘passively’, when seeds or fruits stick to fur, and ‘actively’ when the plant attracts animals to a trophic reward (generally fruits, but sometimes seeds, Fig. 14) with the mobile animal then transporting the seeds and depositing them (often by defecating) some distance away. For the most part, pollination is active with the plant advertising a trophic reward, which may be nectar (a sugar secretion) and/or may be pollen. In the case of pollinators, not only are some species capable of moving pollen but they can do it in a very directed way, transporting it to members of the same species of plant, thereby allowing cross-pollination and cross-fertilization to occur. This specificity (i.e., mobility to a specific, favorable location) occasionally is the case for seed transport, e.g., Clark’s nutcrackers transport seeds to sites that are particularly favorable for the growth of the pines whose seeds they are transporting; certain ant dispersed species bring about movement of seeds to sites (ant nests) that are particularly favorable for seedling establishment (Fig. 14).
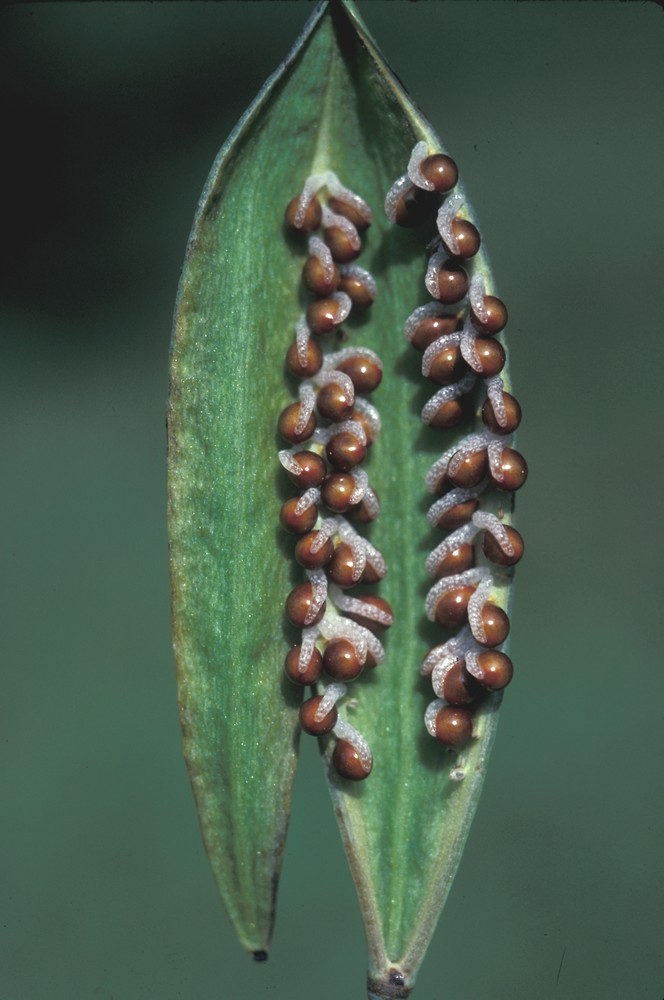
While the common interaction is a ‘quid pro quo’ with food provided by the plant ‘in exchange for’ work by the visitor, occasionally the plant is providing a non-food reward. Some orchids provide specific chemicals that serve as pheromones for the insects that acquire them. The Yucca plant provides not only food but also a shelter for larval yucca moths that develop from the eggs that the yucca moth deposits as it is pollinating the plant. Ficus trees provide food and nesting sites for pollinators.
Two excellent sites that consider these interactions are:
- Yucca moth: https://web.archive.org/web/20211107151421/https://www2.palomar.edu/users/warmstrong/ww0902a.htm
- Ficus: https://web.archive.org/web/20230402002659/http://waynesword.net/pljune99.htm
[Both these links come from an outstanding website (‘Wayne’s Word’) that is an excellent source of botanical information. Take some time to explore it.]
There are a number of examples of ‘cheating’ by both the visitor and the plant. Some visitors can consume the food reward without picking up pollen and consequently without providing the plant with a service. Plants may attract visitors with visual displays but give them no rewards. And, in the case of pitcher plants, the attraction may lead to the demise of the visitor.
‘Protection rackets’ describe a relationship where the plant provides food and another species does work by protecting the plant from herbivores. This relationship is particularly well developed in some Acacia trees and shrubs where the plant not only provides food rewards but also provides nesting spots for colonies of ant defenders. Additionally, while the typical food reward (for pollination, seed dispersal and defense) is nectar, a compound that is cheap to produce, some Acacia species provide more complex food rewards, Beltian bodies, packets that are much more rewarding nutritionally because they contain lipids and proteins. These are much more expensive for the plant to make. An extension of this interaction is the three species interaction of plants, aphids and ants. Aphids are insect herbivores (grazers, sometimes parasites) that tap into the plant’s phloem tissue using their stylet. Since phloem sap has abundant sucrose but scant amino acids, the aphid eats a lot of phloem sap to acquire the amino acids it needs and the excess sucrose is excreted as ‘honeydew’, aka ‘frass’ (insect excrement). Ants have developed a relationship of defending the aphids from predators while acquiring the honeydew that the aphids produce.
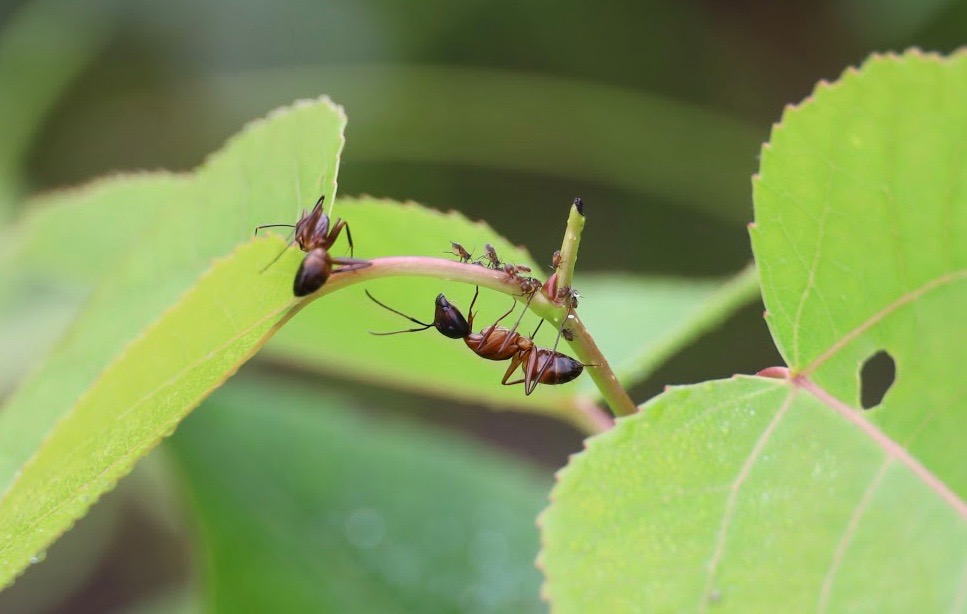
Nitrogen-fixing bacteria do chemical work, the reduction of nitrate to ammonia, for their host plants, in exchange for food and a protected habitat. In nodule forming plants the protected habitat are galls on the roots. The aquatic fern Azolla forms small cavities on the surfaces of leaves that the nitrogen-fixing cyanobacterium Anabaena colonize. This relationship is utilized by rice farmers who encourage the growth of Azolla, a small aquatic plant that lives on the surface of bodies of water. Nitrogen fixed by the cyanobacterium becomes available to the rice when the Azolla dies and is decomposed.
A more ‘one-sided’ work interaction in volves insects acquiring and utilizing chemicals that the plant synthesizes. Monarch butterflies acquire a poison from the milkweed plants that they eat. The plant is grazed by the Monarch caterpillars and gains nothing, but the insect acquires a chemical that deters predation. A similar situation occurs with poison dart frogs. The frogs become poisonous because of the insects that they consume, with yet-to-be-determined plants eaten by the insects providing the specific chemicals. Plants can also be on the receiving end of defensive chemicals as is the case with some fungal endophytes (parasites) who produce toxins that can affect herbivores.
In a number of interactions the ‘work’ is the production of a particular structure (note that producing any biological structure requires material and energy). For example, vines utilize the structure of other plants and thereby avoid the costs associated with producing structural cells (sclerenchyma fibers) that are needed to produce a rigid stem that can withstand the forces of gravity and wind. Another example involving plants are what are called ‘hemiparasites’. These are plants that attach to the roots of other plants, connecting to the xylem, not the phloem. Hence it is not a trophic relationship, food is not transferred from host to ‘parasite’, rather the ‘parasite’ is utilizing the structure produced by its host, the root system, to acquire water and minerals. These move passively from the host’s roots into the stem of the hemiparasite.
At least some of the benefits of mycorrhizal associations may involve a similar type of relationship, with the fungus providing structure to explore the soil to acquire water and nutrients. However, in mycorrhizae the plant fosters the relationship by providing food for the fungus. In endomycorrhizae (vesicular-arbuscular mycorrhizae) that involve Glomeromycota fungi, the arbuscules can be considered a type of haustorium that facilitates transfer of food from the host plant to the fungus. In ectomycorrhizae there are no arbuscules and but the plant ‘leaks’ sufficient food to sustain the fungus. In both types of mycorrhizae the fungus does additional ‘work’ besides simply producing structure. The fungi are producing enzymes that mobilize mineral elements that can be them to their host.
Finally, some of the well-known ‘symbiotic’ relationships involve structural features. One might consider the role of coral animals and lichen fungi to be providing services (structure) for the dinoflagellates and algae that live inside them.
Further Reading and Viewing
- “Botany Blog – Dispersal of Trillium seeds by ants.”
- “Australian Museum – Herbivory: eating plants.”
- “Mycoloop: chytrids in aquatic food webs” by Maiko Kagami. Chytrids in food webs.
- “The Battle Below – Saprophytes and Mycorrhizal Fungi Compete for the Same Substrate.” Fungal interactions below ground.
- “Fungal Haustoria Absorb Nutrients from Living Plant Cells” by Helga George.
- “How radioactive carbon from nuclear bomb tests can tell us what parasitic orchids are eating.” What are parasitic orchids eating?
- “Parasitism finds many solutions to the same problems in red algae (Florideophyceae, Rhodophyta)” by Jillian M Freese. Red algal parasites.
- “The Microbial World: Biotrophic plant pathogens” by Jim Deacon
Media Attributions
- Wolf © Arturo de Frias Marques is licensed under a CC BY-SA (Attribution ShareAlike) license
- Red Crossbills © Elaine R. Wilson is licensed under a CC BY-SA (Attribution ShareAlike) license
- Goldfinch © Ken Thomas is licensed under a Public Domain license
- Japanese beetle © Zech Smith is licensed under a CC BY-SA (Attribution ShareAlike) license
- leaf hopper © JJ Harrison is licensed under a CC BY-SA (Attribution ShareAlike) license
- Weevil © Sarefo is licensed under a CC BY-SA (Attribution ShareAlike) license
- Dodder Vine © Michpass is licensed under a CC BY-SA (Attribution ShareAlike) license
- Galls © Jmeeter is licensed under a CC BY-SA (Attribution ShareAlike) license
- Red bog-moss (Sphagnum capillifolium) © Andrew Curtis is licensed under a CC BY-SA (Attribution ShareAlike) license
- Bog © cjuneau is licensed under a CC BY (Attribution) license
- Osprey © Rhododendrites is licensed under a CC BY-SA (Attribution ShareAlike) license
- Hummingbird © Lorie Shaull is licensed under a CC BY-SA (Attribution ShareAlike) license
- Fruits: Sanguinaria canadensis © John Lynch © 2022 Native Plant Trust with permission is licensed under a All Rights Reserved license
- Ants crossing